Researchers at IISc have been investigating various properties of these unique materials
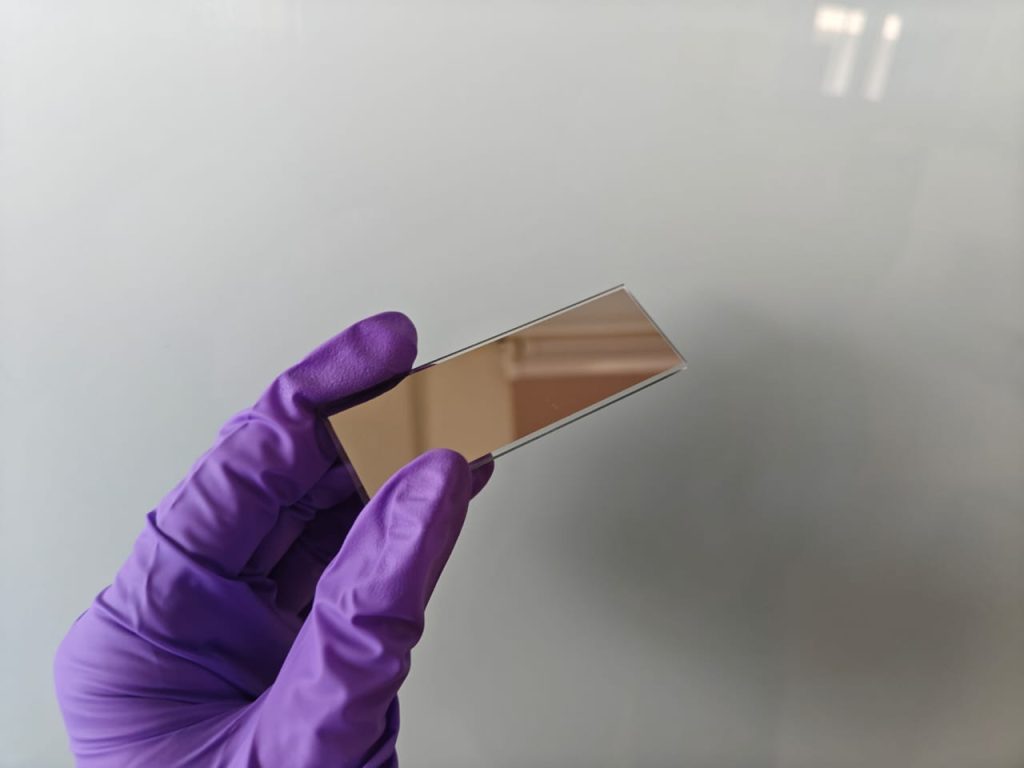
In today’s fast-paced technological age, we are continuously generating data, from everyday camera clicks to extensive healthcare databases. These huge amounts of data have created an increasing demand for faster and more efficient data storage solutions. Current storage solutions like HDDs and SSDs are expensive, non-recyclable and unable to keep up with the rapid pace at which data is generated. This is why scientists, including those at IISc, have turned to chalcogenides.
Chalcogenides are alloys containing predominantly chalcogens – elements that belong to the oxygen family (the sixth group) on the periodic table. Chalcogenides can be crystalline or non-crystalline (also called amorphous or glassy) in nature. Chalcogens usually have a high resistivity and are considered poor conductors. However, when combined with different metals/semimetals, their structure and resistivity can be manipulated, making them conduct electricity. Chalcogenides can also be tailored to change their light reflection capability and sensitivity to different electromagnetic rays.
“Combining chalcogens with group IV elements like germanium and silicon gives them very interesting properties,” explains S Asokan, Professor at the Department of Instrumentation and Applied Physics, IISc.
Their special properties make chalcogenides, especially glassy chalcogenides, very versatile with uses in optical bioimaging, solar cells, light-based catalysis, lithium-ion batteries and more. Significantly, chalcogenide glasses have revolutionised optical memory storage using CDs and DVDs. By using an amorphous chalcogenide layer that changes reflectivity under laser light, a large amount of data can be encoded and retrieved easily and rapidly. Although these CDs and DVDs are now largely obsolete due to the emergence of more efficient and higher-capacity solutions like solid-state drives (SSDs) and cloud storage, amorphous chalcogenides continue to be useful in Phase Change Memory (PCM) technologies.
“One of the most interesting properties of glassy chalcogenide materials is what is known as phase change,” says Asokan. Chalcogenide materials can switch between a disordered amorphous state (OFF state) and an ordered crystalline state (ON state) through the application of electric field or light, which represent the digital binary zeros and ones. Unlike traditional methods which use electrical voltage, chalcogenides can use electrical, heat or light pulses for this switching. This enables rapid and reliable data writing and reading which can be used to create PCM technology.
Asokan’s lab was one of the few groups in India to work on glassy chalcogenides in the 1980s. At that time, the electrical switching behaviour of glassy chalcogenides was not well understood, because it does not follow the basic Ohm’s law. “Ohm’s law states that if the voltage increases, the current should increase. If you look at the I-V (current vs voltage) characteristic of these glassy chalcogenide materials at some threshold voltage, corresponding with a threshold current value, they start exhibiting a negative resistance – with the increase in voltage, the current decreases,” explains Asokan.
Asokan’s group studied the I-V characteristics of glassy chalcogenides to understand them better. At that time, they had to build their own systems with constant current supply and many other devices from scratch. They studied the state of local structural order in the amorphous chalcogenides as these influenced their properties and switching behaviour. Over two decades, they also looked into multiple properties of chalcogenides formed from different compositions of metals/semimetals and chalcogens to understand how to tune them to achieve desired properties. Later, they shifted their attention to the optical properties of chalcogenides and other materials so that they could be used for stress-sensing applications.
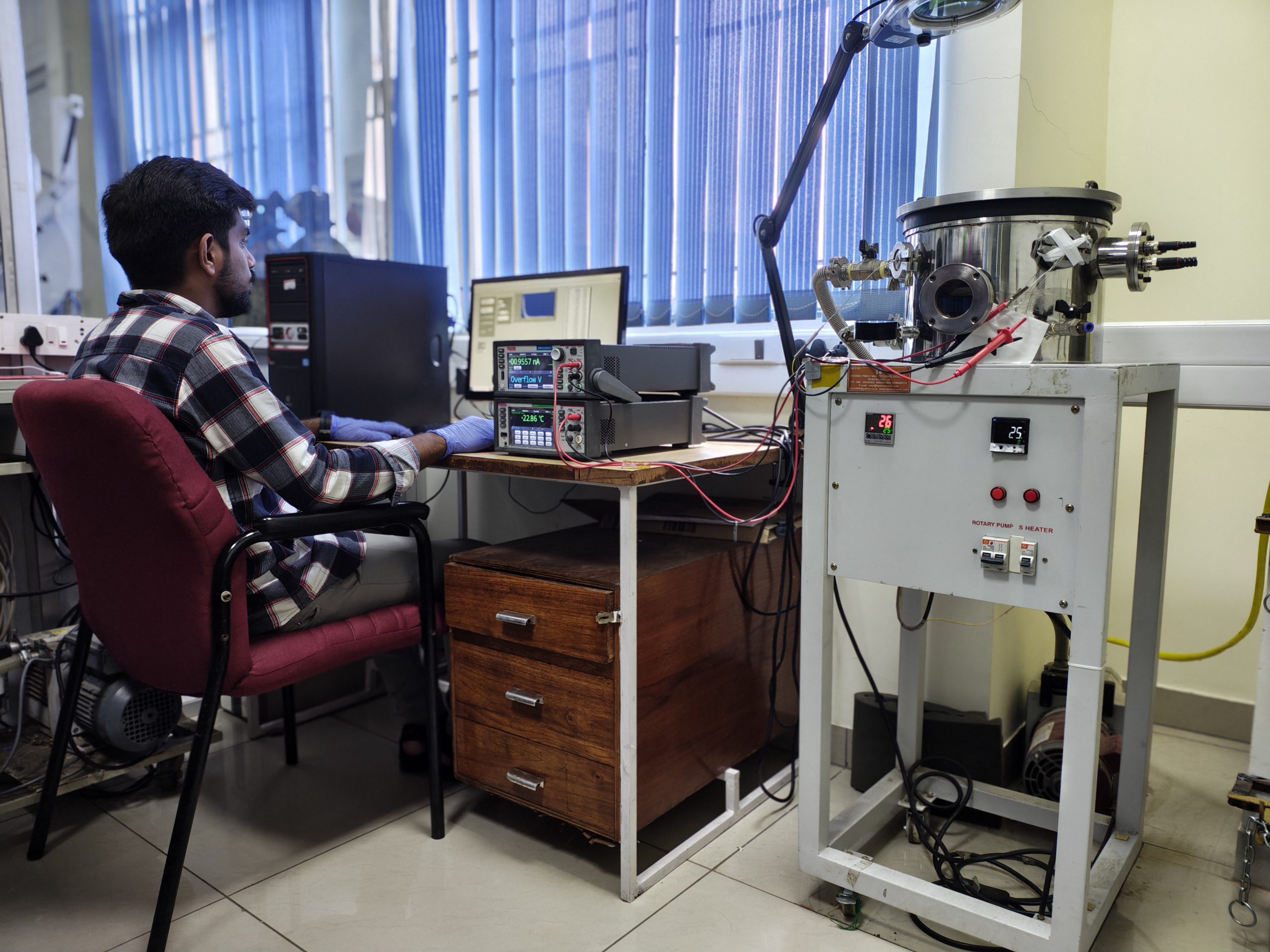
The switching behaviour of amorphous chalcogenides formed the basis for research into how these materials can be used for memory storage. Thin films of chalcogenides can be toggled like on/off switches between amorphous and crystalline states to store information in the 0 and 1 format, respectively, by using different cooling rates. Slow cooling can result in them becoming a highly ordered crystalline state, whereas rapid cooling results in them forming a disordered amorphous state. This allows for “writing” data on the chalcogenide film. To “erase” this data, the chalcogenides are heated again to bring them back to their original state. However, sometimes, continuous current supply may be required to retain the written data, resulting in a RAM-like memory.
K Ramesh, Principal Research Scientist in the Department of Physics at IISc, has also been studying the capabilities of amorphous chalcogenides for phase change memory (PCM) applications. “The contrast in electrical resistance between the high resistive amorphous state and the low resistive crystalline state is ∼103. Importantly, they are non-volatile and have high scalability, endurance, shorter write and read times, and high data retention capability. Hence, they are useful for high-density data storage and memory applications. They are also being explored as non-von Neumann computing for neuromorphic computing applications,” says Ramesh.
His team works on improving their crystallisation speed, endurance and data retention capabilities. They zeroed in on GST as a base material – an alloy of two parts of germanium, two parts of antimony and five parts of tellurium (Ge2Sb2Te5), a commonly used chalcogenide in phase change memory. “In GST … phase change between amorphous and crystalline states (WRITE) is very fast, within a few nanoseconds, and re-amorphisation (ERASE) is in picoseconds,” Ramesh explains. His group looks at the atomic size effect on phase change properties. Adding different-sized atoms distorts the structure, allowing fast crystallisation and improving their phase change, switching and memory properties. Controlled crystallisation to achieve multilevel data storage is also one of their group’s goals. This makes them better at high-density data storage and retrieval.
However, teasing out chalcogenide properties is a complex process with multiple steps, explains Rohit Saini, a PhD student in Ramesh’s lab. The first step is producing the chalcogenide alloy. Researchers must first mix different materials in the required ratios and melt them under a high vacuum. This melt is then homogenised over a long time and quenched to create the required chalcogenide alloy. Afterwards, the prepared alloy is deposited onto a desired substrate by physical vapour deposition. “We make the devices in the sandwich geometry and study their I-V and switching characteristics,” he says.
To study the material’s atomic structure, scientists first use X-rays. Then, they give the material different electrical or optical stimuli to gauge its resistivity, temperature, voltage and other properties. The lab also collaborates with Shankar Kumar Selvaraja’s research group to design and develop low-loss PCMs and integrate them with neuromorphic photonic circuits.
Because of their unique properties, chalcogenides also offer great promise in industry, defence and space applications. For example, Ramesh’s lab prepares bulk chalcogenide glasses of large diameter to make infrared transmitting lenses for night vision and anti-tank guided missile (ATGM) applications in collaboration with the Defence Research and Development Organisation (DRDO). These glasses can detect infrared light that is not visible to our eyes and allow people to see at night.
Over the next few years, Ramesh’s lab seeks to work on chalcogenide selector materials called Ovonic Threshold Switch (OTS). OTS devices can prevent crosstalk of data between two neighbouring cells in a storage device. This would result in lower data loss and protect it from corruption, a vital step towards more efficient data storage.