Naga Phani Aetukuri’s lab studies material interfaces to develop better batteries
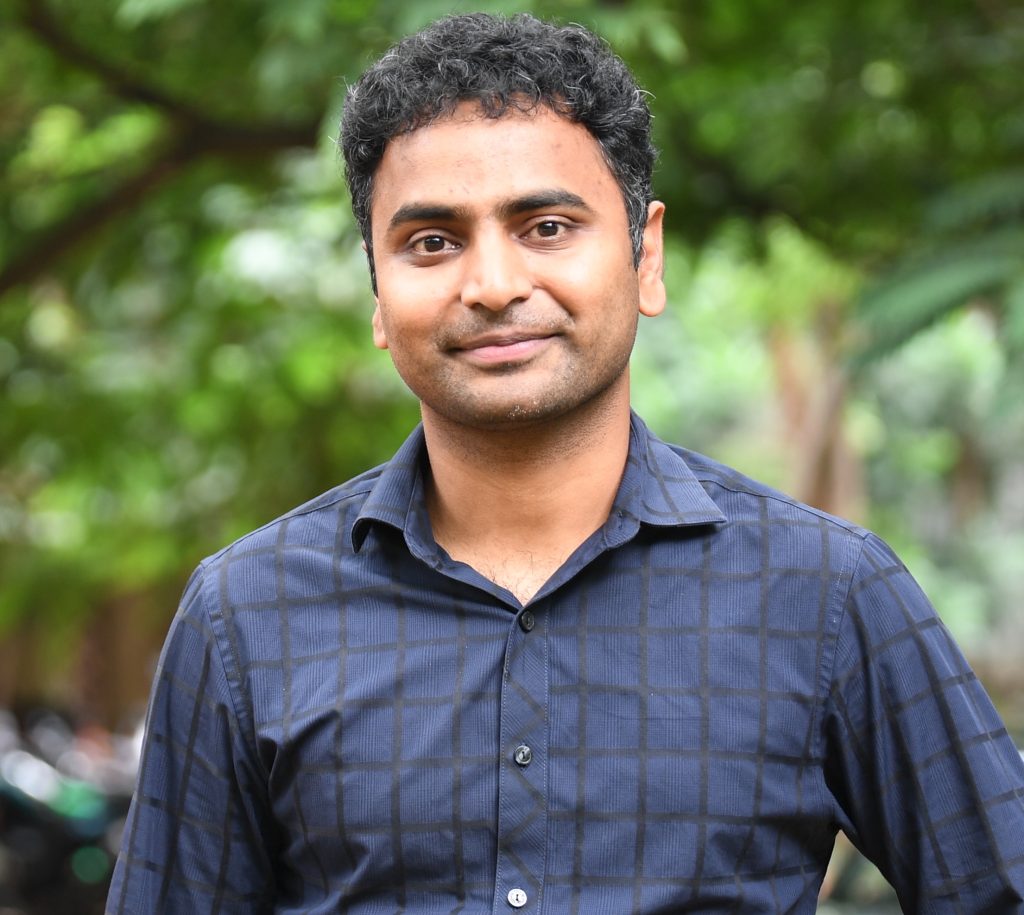
Electric vehicles are all the rage today, thanks to the lithium-ion batteries that power them. These batteries are sought after because they are durable, safe, and can store a lot of energy. But how exactly do these batteries work, and how can we improve them? These are the central questions that Naga Phani Aetukuri’s lab at the Solid State and Structural Chemistry Unit (SSCU) focuses on. The answers lie in tracking the movement of tiny charged particles across material interfaces.
Lithium-ion batteries are simple – they have a cathode (positive electrode) made of a transition metal compound, an anode (negative electrode) made of graphite, and an electrolyte which acts as a medium or channel between the two. When the battery is in use and discharging, lithium ions move from the anode to the cathode via the electrolyte, leaving behind electrons that can move and generate current in the circuit outside. When the battery is being charged, the reverse happens. Using lithium as the anode material increases the energy density of the battery – this means that for the same weight, a phone’s charge will last longer, a car can drive a farther distance, and the battery itself can cost much less than those made of other materials.
Traditionally, the electrolyte is a liquid solution of a lithium salt. But scientists are now turning to solid materials like ceramics instead, leading to the rise of solid-state lithium-ion batteries.
Many properties of a material can be tweaked by changing its composition and the way it is made. But its ability to store energy is driven by what the interface between the electrode and electrolyte looks like, what chemical reactions happen there, and how charged particles (electrons or ions) move across the interface. These ‘interfacial’ interactions dictate how useful the battery can be and if it is likely to suddenly stop working. Phani’s lab seeks to understand what happens at these material interfaces – which phenomena limit the energy storage, and what can be done to improve performance.
Passion for photovoltaics
Phani first became interested in energy research when he was doing his Bachelor’s at NIT Warangal. While pursuing an internship with faculty member Prathap Haridoss at IIT Madras, he developed an interest in photovoltaics, specifically core-shell quantum dots. Quantum dots are tiny particles that can absorb and emit light at specific wavelengths. The energy absorbed by these quantum dots can be tuned easily by changing their size, allowing them to harness a large part of the solar spectrum. Quantum dots can convert this light energy into other forms, making them a promising material in energy research.
Prathap encouraged Phani to apply for a PhD in the USA; the latter heeded his advice and joined Stanford University. “Unfortunately, back in 2006 when I was a grad student, everyone wanted to work in photovoltaics, and so I couldn’t get into the research groups that I wanted to,” Phani recalls. “The opening I had was to work with Jim Harris and Stuart Parkin [researchers at Stanford and IBM Research], on oxide heterostructures.” Oxide heterostructures contain interfaces between two materials having different properties and are often used in electronic devices. Though this did not entirely align with Phani’s interest, he was not deterred.
Towards the end of his PhD, Phani was working on interfaces between transition metal compounds and ionic liquids. He then received an opportunity to get back to energy research, specifically metal-oxygen batteries, as a postdoctoral researcher at IBM Research. These batteries use oxygen from the air to produce electricity by oxidising a metal. Despite having no prior experience in electrochemistry, Phani took up the challenge as it offered him a chance to return to energy research.
By the time Phani returned to India and joined IISc in 2016, he had developed a strong passion for solid-state batteries. But only a handful of people were working in the area. “I saw that there was merit in trying to understand lithium deposition in solid-state systems and we started our work there,” remarks Phani.
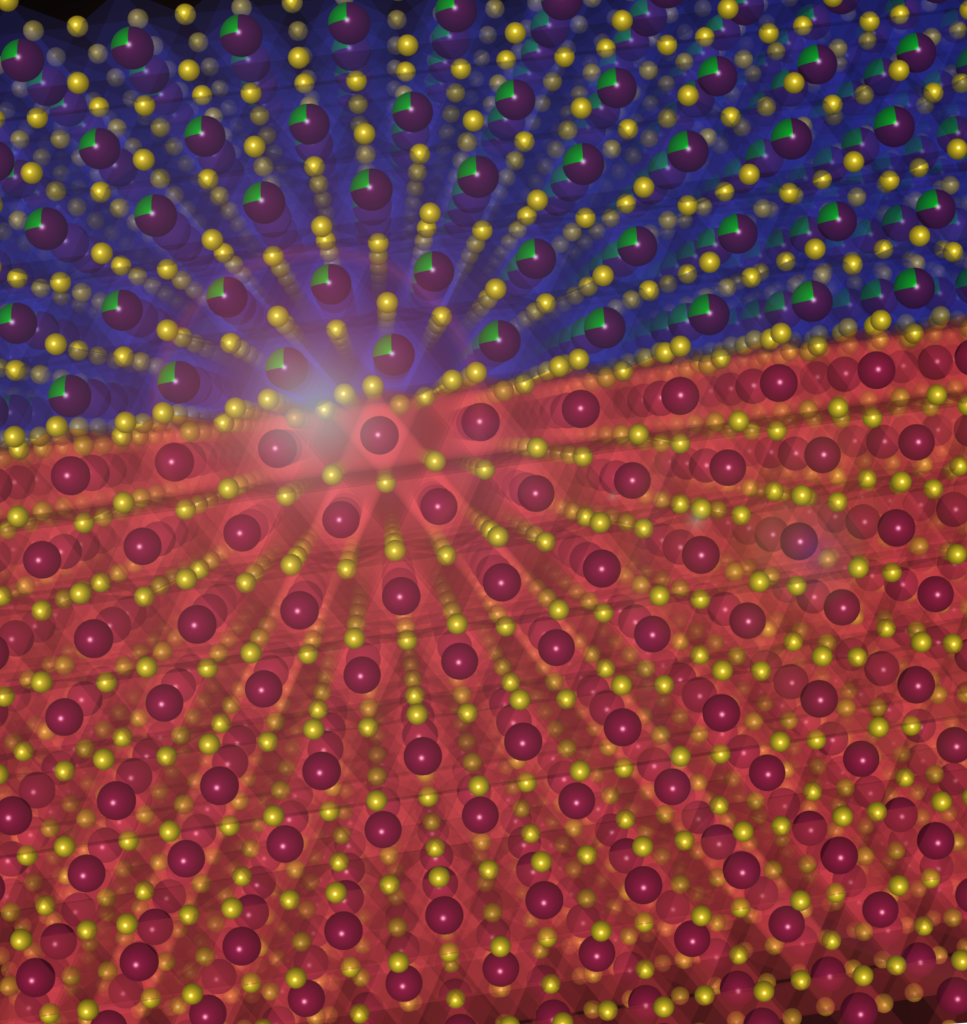
Probing battery failure
For decades, scientists have grappled with a major concern about liquid lithium-ion batteries. Thin needles of lithium called “dendrites” can grow and stretch from the anode to the cathode, leading to a short circuit and increasing the risk of fires. Twenty years ago, some scientists suggested that using a solid ceramic electrolyte instead would stop the lithium dendrites from penetrating the electrolyte. This was what gave birth to solid-state batteries – but there was a catch.
“Lithium is soft; a ceramic electrolyte is hard. Think about anything squishy, and pushing it against something hard like glass. [People thought that] there’s no way the squishy thing is going to penetrate glass,” Phani explains. “However, every experiment that was done showed that lithium penetration through solid electrolytes occurs at current densities much smaller than where it was occurring in liquid electrolytes.”
This puzzled researchers for several years, and Phani’s group decided to probe why. What they found is that every time a lithium ion moves from the anode into the electrolyte, it leaves behind an empty space called a vacancy. This happens all along the lithium ceramic interfaces, and all the vacancies tend to come together to form a huge space called a void. The uneven distribution of voids causes lithium to deposit in some regions of the anode more than others, leading to dendrite growth.
Phani’s group has shown that by using materials which do not alloy with lithium, we can decrease the rate of movement of lithium, which prevents the vacancies from coalescing into huge voids. In subsequent work, Phani’s group also figured out how mechanical potential – the pressure generated due to excessive lithium deposition in a tight space – and the curvature of grains in the ceramic play a role in lithium dendrite growth.
“We can now develop batteries with much higher current densities, which would hopefully be relevant for applications,” says Phani. “That is only possible because we were able to understand the microscopic mechanistics of what happens in these systems and at interfaces.”
Beyond batteries
A part of Phani’s group is also working on oxide heterostructures, the area that he had explored during his PhD. When you put together two different materials, the electrons on either side of the interface experience a different ‘chemical potential,’ creating an internal electric field which forces the electrons to move from one material to the other – just as a ball rolls down from a height. Unlike an external electric field, this does not change the structure of the material, but the charge carrier (electron) concentration shoots up, which increases its ability to conduct current. Phani’s lab has been trying to use this principle to increase carrier concentration in vanadium oxide – an insulator – to make it behave more like a metal, in an effort to create a high-temperature superconductor.
Phani’s lab is also working on organic semiconductors, which contain a solid medium made up of organic polymers. While most scientists view these materials as analogues of inorganic semiconductors, Phani argues that they are actually closer to electrolytes in how they behave. Some of his published work highlights how theories used to describe charge conduction in electrolytes can also be used to understand organic semiconductors.
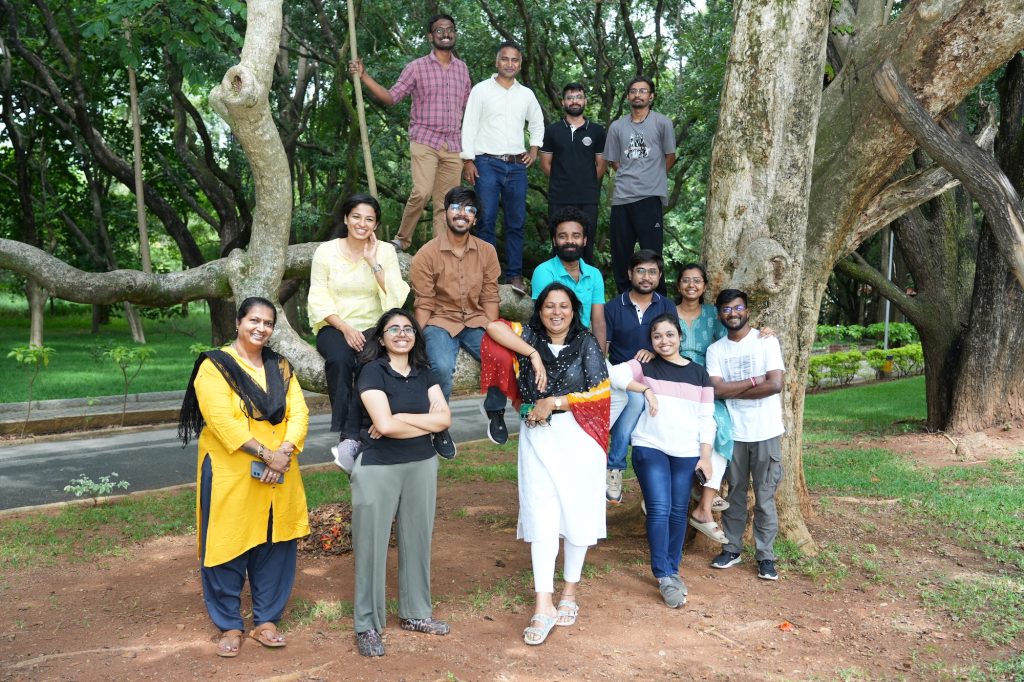
When he’s not in the lab, Phani enjoys playing cricket and chess, and spending time in his garden taking care of his plants. “That kind of calms me down,” he explains.
Phani also has his eye on electrochemical manufacturing. Traditionally, industrial processes have relied on thermal energy, but that has changed with the emergence of renewable sources. “If we take ourselves back to about 500 years ago, it was easy to burn coal; today, it’s easier to install a solar cell,” Phani explains. “So, how can we utilise the abundance of electricity? We are hoping to develop new processes which can address the issue of CO2 emissions from fuels, and at the same time use a fuel source that costs nearly nothing.”
Phani strongly believes that research shouldn’t happen in silos. “It’s one thing to discover something for the first time – it’s more important to ask how your work can be utilised by a larger section of people. Whatever you do needs to have a broader implication for science in general.”