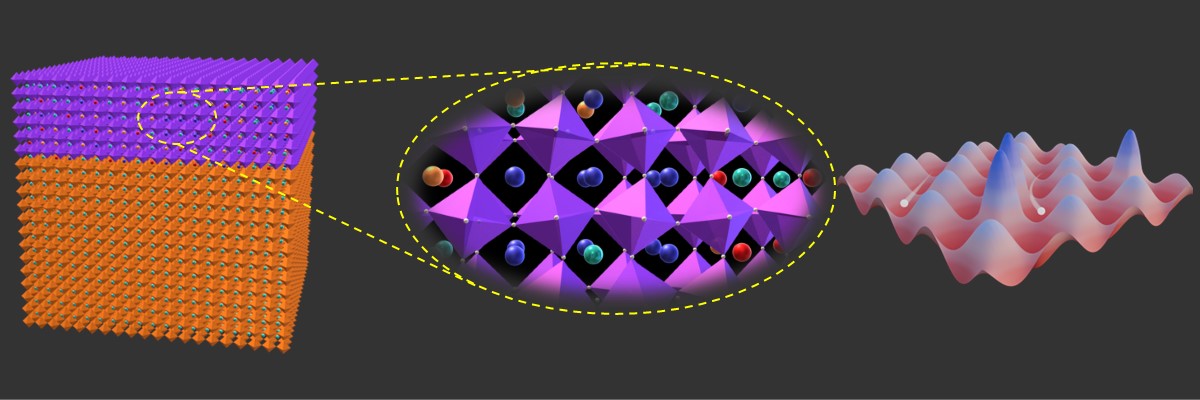
In recent years, high entropy alloys (HEAs) are becoming increasingly popular. HEAs are formed when five or more elements are mixed to form alloys with high configurational disorder. These materials exhibit exceptional material strength and offer promise for applications in areas like aerospace and automotive industries where high performance and durability are critical.
Beyond mechanical strength of HEAs, the high entropy recipe has been incorporated in the family of quantum materials since 2015, leading to the emergence of unique electronic, magnetic, and optical functionalities credited to unique locally broken symmetries offered by disorder. However, to trace the origin of these properties and explore their practical utility, it is crucial to grasp the underlying fundamentals. For instance, almost all high entropy ceramics are either semiconductors or electrical insulators. A key question unanswered so far is how electrons traverse in such extremely disordered systems.
In a new study published in Advanced Materials, researchers at the Department of Physics, led by Srimanta Middey’s group, with collaborators at DESY (Germany), Advanced Photon Source (USA), and East China Normal University (China), investigated a rare high entropy oxide –(LaPrNdSmEu)0.2NiO3 – which exhibits a temperature-dependent metal-to-insulator transition. To study this material, they used several complimentary techniques, including synchrotron X-ray diffraction, X-ray absorption spectroscopy, hard X-ray photoemission spectroscopy, and scanning transmission electron microscopy.
Interestingly, in thin film geometry under in-plane elongation, they reveal that even with the seemingly random nature at the rare earth sites in the material, there is an “order within the disorder” emanating from nanoscale atomic clusters. Although these create roadblocks, electrons travel along the metallic matrix to avoid these insulating islands, thus enhancing the resistivity of the metallic phase. On the contrary, in-plane compression overrides the tendency towards this electronic phase separation, leading to an exotic, fully metallic state at low temperatures.
This opens up avenues for carefully controlling the compositional complexity and employing strain as a fine-tuning parameter to design functional properties of high entropy quantum materials.