Scientists are studying the myriad roles of a muscle protein
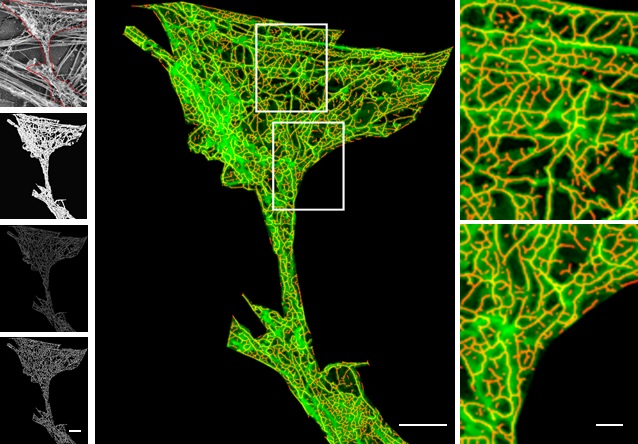
“To see them [muscles] contract for the first time, and to have reproduced in vitro one of the oldest signs of life, motion, was perhaps the most thrilling moment of my life,” said Albert Szent-Györgyi, a Hungarian scientist, in 1963, describing his discovery of actin’s role in muscle contraction.
In the decades since, scientists have pieced together the picture of how this protein shapes, moves and directs life-or-death decisions in living cells. Researchers across disciplines have been decoding the nuances of actin function and behaviour. This is important because actin is also associated with a variety of diseases ranging from muscular disorders and cardiac myopathy to neurological conditions such as Alzheimer’s disease.
Actin acts on multiple processes in our cells. Its most well-known role is in making our muscles move. What is lesser known, however, is its contribution to shaping neuronal signalling and driving cancer cell growth. To study these processes, scientists at IISc and elsewhere are using a variety of approaches, from microscopy to maths equations.
Wearing many hats
Actin is a polymeric protein made of individual globular units strung together like beads on a necklace. What makes it unique is its versatility in structure and function.
A thin belt of actin called ‘cortical actin’ is present around the cell membrane to hold the cell’s shape. The cell membrane is also connected to rope-like actin structures called stress fibres. They tether the cell to the tissue at the base, like ropes holding down a tent.
Besides tethering cells, actin also enables cells to move. When immune cells are hunting down harmful microbes, actin forms projections called lamellipodia in front of the cell. Lamellipodia are sheet-like structures made of branched actin, with which cells reach out to form attachments as they move.
Actin owes its versatility to regulatory proteins that bind to it and shape its function. A protein called cofilin, for example, chops down actin filaments by removing individual units from the polymer, while another protein called arp2/3 creates branching patterns. “Actin is like a Lego piece that can be purposed to any structure that has to be generated, and that too in a dynamic fashion,” says Sudha Kumari, Assistant Professor at the Department of Microbiology and Cell Biology, IISc.
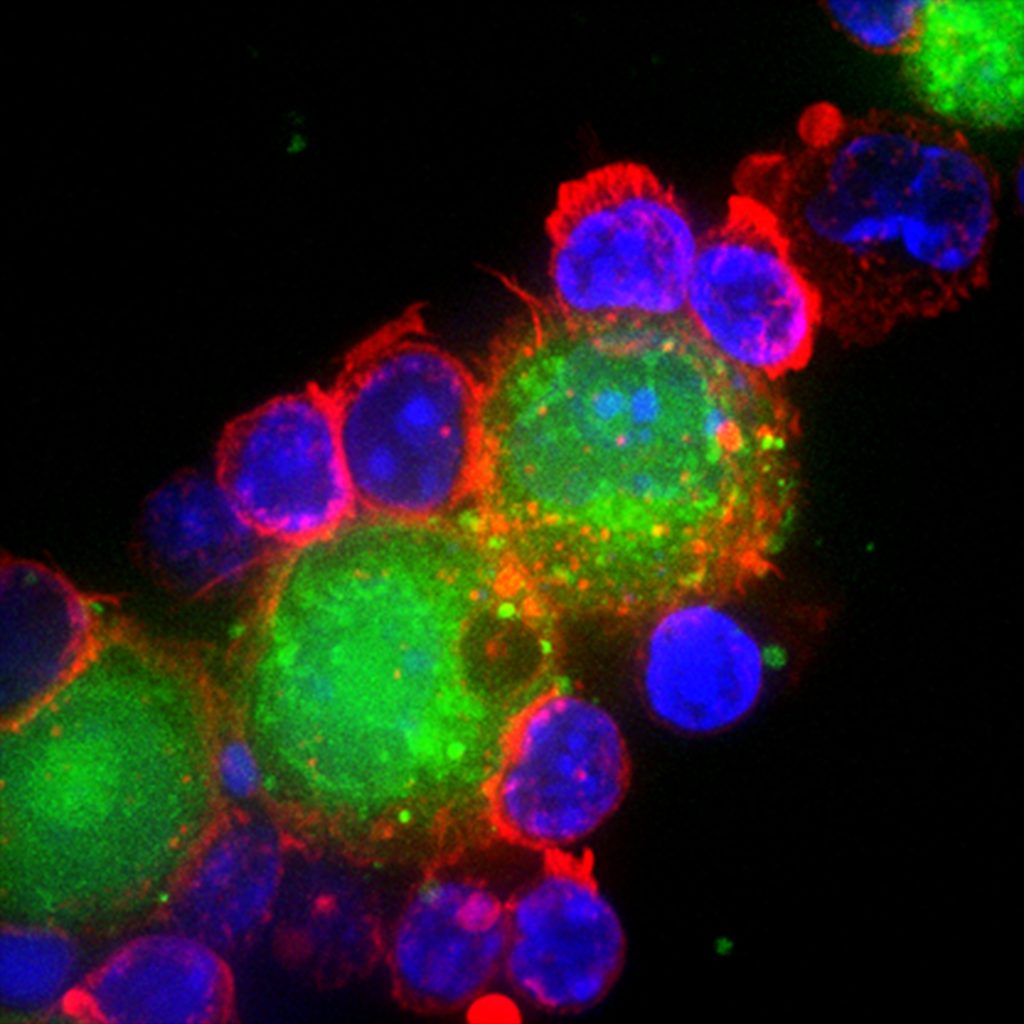
From structures to signalling
Actin’s role is not restricted to maintaining shape. Scientists now know that it is also involved in cell signalling during events such as T cell activation. If a T cell encounters a pathogen while surveilling our body, it becomes activated and forms immunological “synapses”. These synapses are regions where proteins of immune cells interact with proteins of the pathogen. During T cell activation, actin forms different structures that serve as sites for other proteins to bind, which activates specific signaling pathways.
From immunosurveillance to migration and activation of immune cells, actin has the remarkable ability to adapt its structure to aid diverse processes in the cell. “We are trying to understand how actin specifically participates in one process versus the other,” says Sudha.
Besides immunological synapses, actin is also crucial to the functioning of neuronal synapses. These are the tiny gaps where electrical and chemical signals are relayed from one neuron to the next. Deepak Nair, Associate Professor at the Centre for Neuroscience, is studying the role of actin in shaping these synapses.
Neurons have branched structures called axons and dendrites that allow them to send and receive signals. The dendrites possess small, mushroom-shaped, actin-rich structures called dendritic spines, which serve as specialised compartments for receiving signals. The molecular composition and shape of these spines are crucial for cognitive processes such as learning and memory, and their disruption is linked to the onset of neurological diseases.
Actin networks sculpt dendritic spines into diverse branching patterns – some resemble palm trees with broad swathes, others look like gulmohar trees with umbrella-shaped canopies, while some take on shrub-like forms with intricate branches and sub-branches. These branching patterns influence the neuron’s capacity to encode and decode synaptic signals. “It is in these spines that most of the molecular changes associated with synaptic plasticity get kickstarted,” explains Deepak. Notably, these structures are damaged in neurodegenerative disorders such as Alzheimer’s disease. A few years ago, Deepak’s group, in collaboration with the group of Vijayalakshmi Ravindranath, founder director of the Centre for Brain Research, found that upon correcting actin organisation, they could slow down or partially rescue the progression of Alzheimer’s disease in mice models.
Actin also shapes the structure of synapse itself. Neural synapses come in different configurations – they can be mushroom-like or fork-shaped or form a shrub-like ‘stubby synapse’. Mushroom-shaped synapses are associated with higher learning and fork-shaped synapses are associated with forgetfulness. To study how actin branches out to create these different synapses, Deepak’s lab uses a super-resolution microscope to study actin in action. They then use AI-based pattern recognition tools to characterise different actin structures.
“The beautiful thing about actin is that it self-organises into different structures. This not only has therapeutic uses, but is also a design blueprint for engineers to mimic,” says Deepak.
Seeing without changing
Because of its diverse roles, scientists are keen on tracking actin under the microscope. However, the very act of observing actin can alter its behaviour. To visualise actin under a microscope, researchers use chemicals with fluorescent tags that bind to actin. But these tags can modify actin’s structure and function.
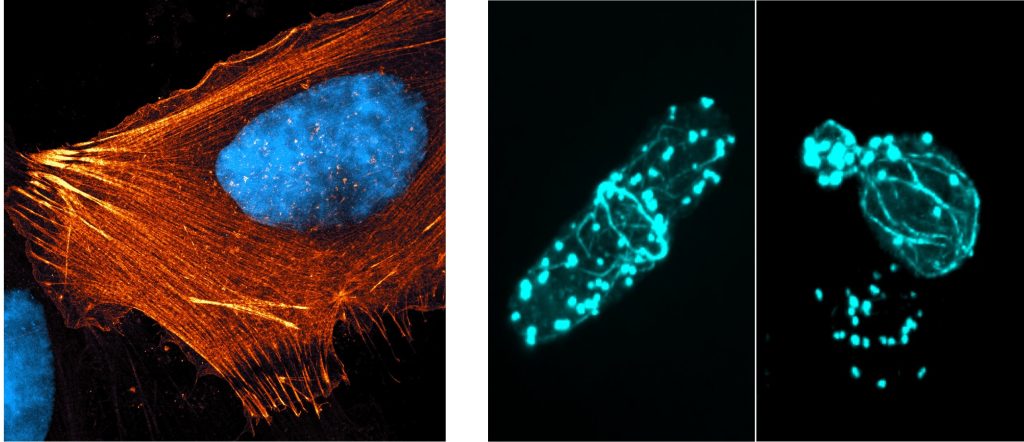
Images taken with an Olympus SpinSR confocal microscope in Saravanan’s lab. Stained structures represent actin filaments and diverse network types of these different species
(Image courtesy: Anubhav, Nishanth, Saravanan, Sanjana, Sucheta, Sudharshan)
“If you put a tag at the wrong position in actin, it affects polymerisation and the actin filament dynamics can change,” explains Anubhav Dhar, a PhD student in the lab of Saravanan Palani, Assistant Professor in the Department of Biochemistry. To address this, researchers from Saravanan’s group collaborated with Koen van den Dries’ research group at the Wageningen University and Research, Netherlands. They analysed the structure of the actin protein to identify precise sites where tags can bind without disrupting its structure and function. Based on this, they developed an Internal Actin Tagging strategy called ‘IntAct’, where short amino acid sequences are inserted into specific internal positions within the actin protein.
To zoom in further on actin, researchers use a tool called expansion microscopy. In expansion microscopy, the biological sample is physically expanded, like inflating a balloon. The sample is soaked in a hydrogel which absorbs water and swells. However, for the sample to be inflated, it needs to be denatured with heat, which causes the actin probes to fall apart from the actin filaments and then, actin cannot be visualised. To tackle this, Saravanan’s team added an ‘ALFA’ tag in their IntAct probe, which can bind to a small nanobody to which a fluorescent protein is attached. This nanobody probe can bind to the ALFA-tagged actin even after the denaturation and expansion. “After over a decade of the development of expansion microscopy, we are now able to use it to see networks of actin with such unprecedented detail in cells for the first time,” says Saravanan.
Actin in cancer
Sumantra Sarkar, Assistant Professor at the Department of Physics, and his lab use maths models and approaches to study how actin interacts with the cell membrane and affects signaling proteins present on the cell surface. They are particularly interested in its role in cancer cells.
For example, a signalling protein called RAS that gets activated on the cell membrane, has been linked to increased cell division in cancer cells. Sumantra’s team noticed that there was no difference in the amount of RAS in the cell membranes of cancer cells versus healthy cells. But they found that RAS forms a greater number of clusters in cancer cells, due to interactions with actin.
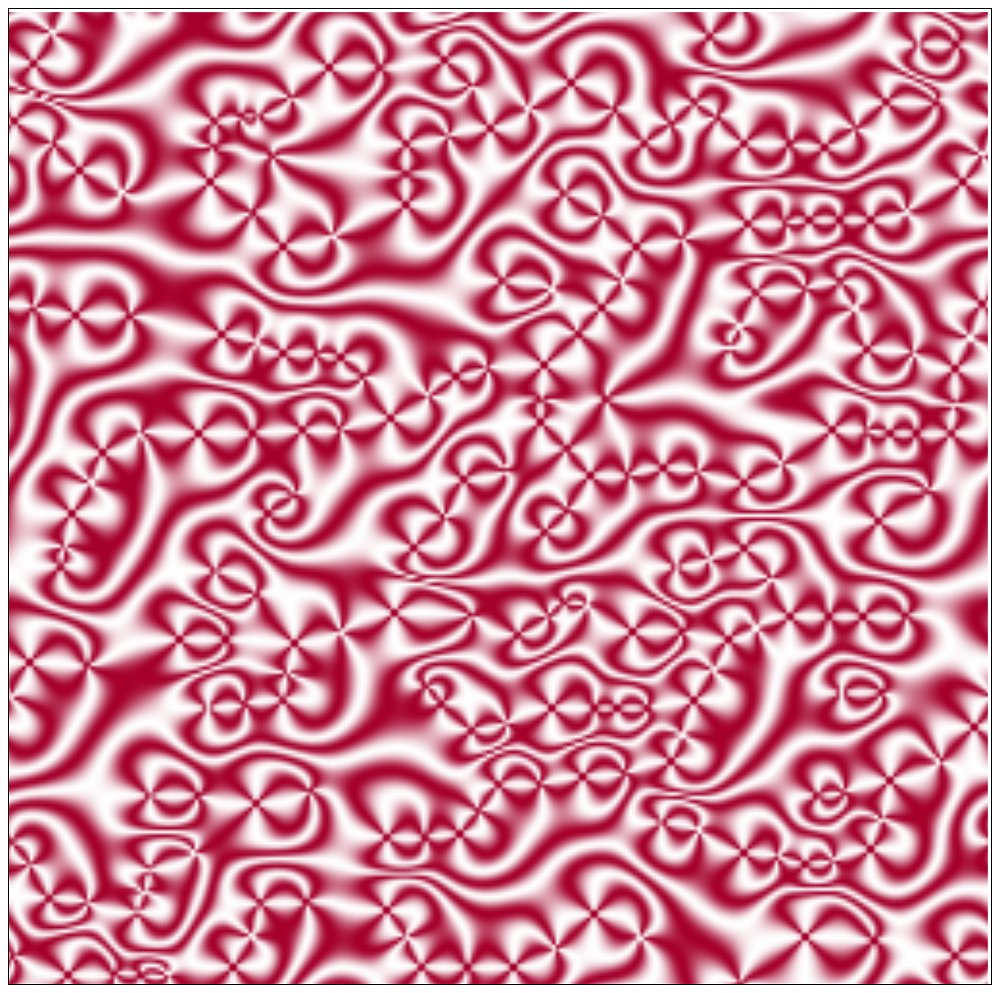
In the cell membrane, actin creates star-like structures called asters, a type of topological defect. At some point, such defects merge and create a self-organised structure to which proteins like RAS can bind and cluster on the cell membrane. These clusters stay for a longer time on the cell membrane.
Sumantra’s team found that cancer cells have a mutation in the RAS protein, which increased cluster formation and survival time. This keeps the cell division signalling pathway turned on for a longer time in cancer cells, helping them divide at a greater rate. “So far, we have studied actin mechanics and biochemistry in isolation,” Sumantra says. But actin’s mechanical properties can directly influence biochemical pathways and vice versa. “Now we’re exploring the feedback between these processes – how mechanics helps chemistry and chemistry influences mechanics.”
Since the time of Szent-Györgyi’s discovery of actin-powered motion, scientists have increasingly realised the growing role that the protein plays in not only healthy cells but also disease. It is becoming clear that actin – or more specifically, its breakdown – is involved in a variety of diseases, from cancer and neurodegenerative disease to muscular disorders.
“Actin is a molecule of target in many diseases, and it opens up different kinds of targeting strategies. Understanding the role of actin in cell growth, cell division and cell death will have major consequences from evolution to health,” says Deepak.