Investigations on air flow in soap bubbles open up new insights and applications
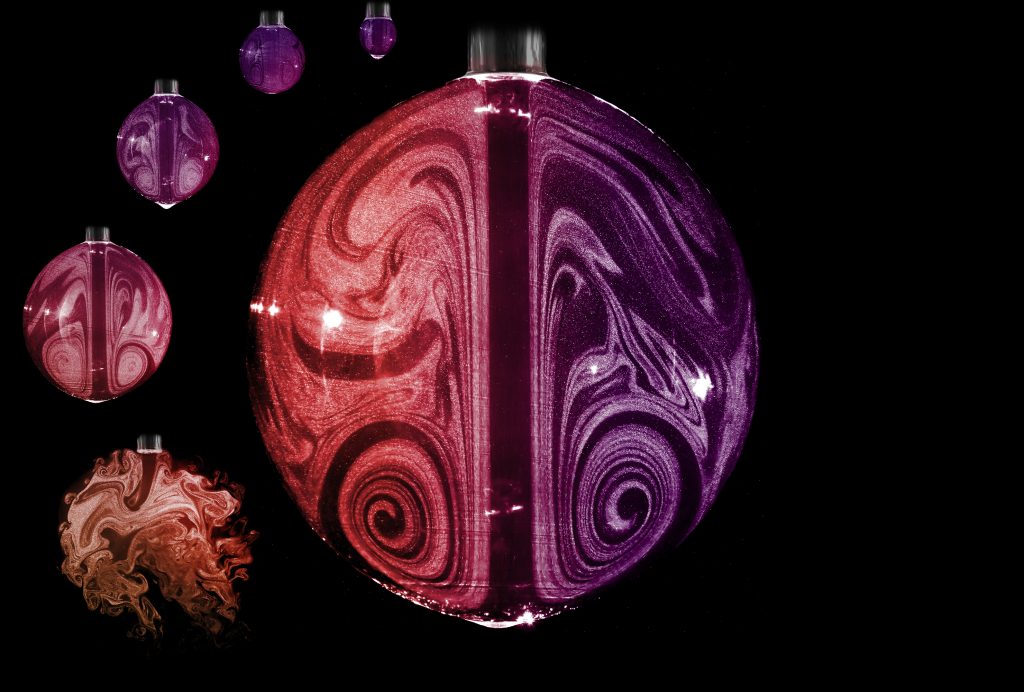
Sometime during late 2023, Saini Jatin Rao was at his favourite tea joint, blowing into his chai as he drank. As his breath split the chai surface, the liquid spinning into the shape of a doughnut, he started wondering: What happens when air flows into a bubble?
Rao rushed to his lab, and along with Siddhant Jain – a fellow research scholar in the Department of Mechanical Engineering – designed a set up to test the idea. They decided to inject a jet of air into a soap bubble in a contained environment, and track how the air flow changed as the bubble grew in size. To counteract the unstable nature of a soap bubble, they built an acrylic chamber with a nozzle to protect the bubble from external disturbances. Once the soap bubble was stable, they introduced small trace particles – olive oil droplets – to follow the air flow inside the bubble. To track the movement of the particles, they flashed a laser beam at the bubble every 30 microseconds. The light scattering properties of the particles provided a clear view of the flow and the patterns created as air rushes into the bubble.
In the beginning, the inside of the bubble was stable. As the bubble grew in size, the air flow started curling up as it interacted with the curved interface of the bubble, forming a vortex. The team tried air flows at different velocities and pressures, to see how the internal flow behaviour changed. When a jet at a higher velocity was introduced, the jet itself and the vortex ring started to quiver but the overall structure of flow remained stable, showing that there was orderliness even when the bubble was moving towards instability – from linear to turbulent flow. Interestingly, this transition, measured by a parameter called Reynold’s Number, was nearly 10 times lower than expected. Depending on the problem at hand, the Reynold’s number for turbulent flows is typically above 2,000-5000; if the number falls below this, the flow is often laminar.
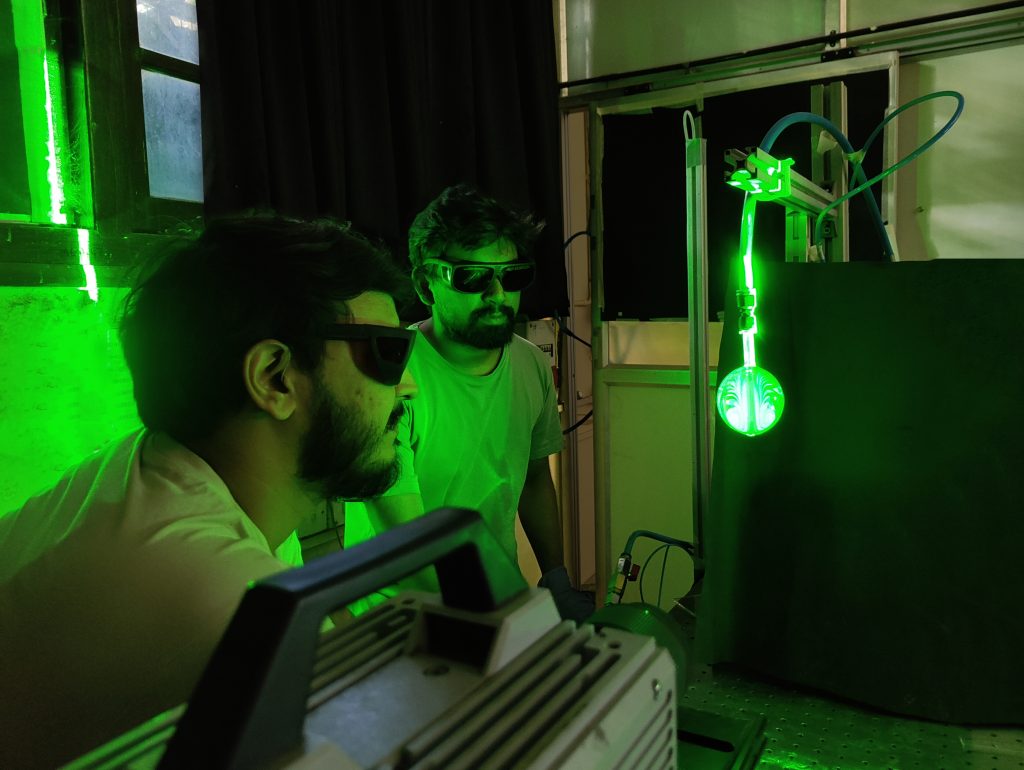
(Photo courtesy: Saini Jatin Rao and Siddhant Jain)
“We started to see instabilities at a much lower Reynold’s number, around 200 to 250. This is something that people have not investigated – low Reynold’s Number turbulence or low Reynold’s number instability. I call it embryonic turbulence,” says Saptarshi Basu, Professor at the Department of Mechanical Engineering, and Rao’s adviser.
Though the experiment has been done on an air-bubble-air interface, the researchers are confident that similar phenomena can be expected in other bubble systems too – air bubbles in water, for example.
Bubbles have long remained a part of human civilization. “We blow soap bubbles all the time,” Basu says. “But little is known about what happens on the inside of the bubble.”
It was this gap in knowledge that motivated the team. The aesthetically delightful patterns – like the mesmerising whirlpools they could see around the inlet jet as the air penetrating the bubble impinges the interface – became a bonus.
Besides providing fundamental insights into air flow, the team believes that there are several practical applications to such explorations, in areas like soft robotics or drug testing, Rao says.
“In micro or milli-scale systems, the problem people encounter is mixing. Because the flow is usually laminar, you don’t get proper mixing because of the low Reynold’s Number. This is an issue in microfluids,” says Basu. “[Our study] offers an insight that if a flow goes into turbulent mode at low Reynold’s Number, then you can have enhanced mixing in systems with microdroplets.”
Another area is testing interactions between disease-causing organisms and drugs. Currently, Rao says, the way that such interactions are tested is by tracking their movement through a long microfluidic network over a long time.
“Here, you do not need that,” Jain says. “You can have individual non-interacting droplets with bacteria and antibiotics at micro levels. You can monitor the growth of bacteria inside these small micro reactor droplets. Because of the toroidal action of the flow, the mixing between bacteria and the drug can be quickly churned like in the body.” This can be particularly helpful in investigating and improving the efficacy of a variety of drugs.