Emerging devices offer diverse applications in biology and drug therapy
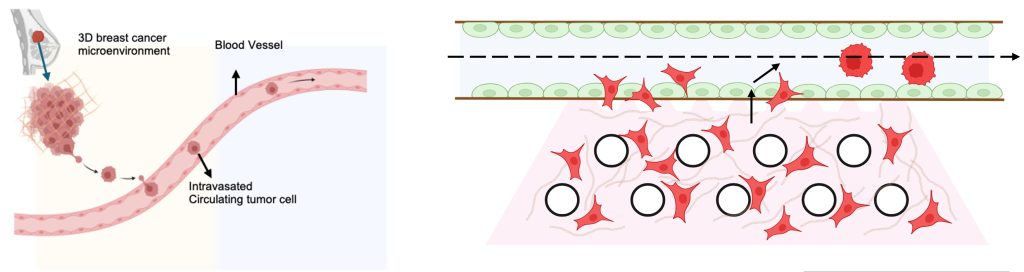
Over the past two decades, organ-on-chip (OoCs) systems have gained traction within the scientific community as an alternative to animal models. These microscopic devices are the size of an average chocolate bar and are made of flexible polymers. These are essentially artificial scaffolds for biological cells to attach and grow just as they would inside the body.
OoCs offer many advantages when compared to 2D or 3D cell culture systems. They can better mimic the tissue microenvironment within the human body. They are also flexible in design and can be adjusted to study any tissue like the lung, heart or blood vessels. They can even be personalised to study an individual’s cells for diagnostic and therapeutic applications.
About four years ago, Ramray Bhat, Associate Professor at the Department of Developmental Biology and Genetics (DBG), noticed that most of the OoCs in India were exported from Europe and the USA. He was tinkering with the idea of developing an in-house OoC system at IISc. Ramray’s research involves studying tissue structure and function, and their role in cancer spread. He wanted to understand how cancer cells intravasate – move from their place of origin and enter the circulation so as to eventually reach other organs and settle there.
He approached Prosenjit Sen, Associate Professor at the Centre for Nano Science and Engineering (CeNSE), and the two discussed the idea of designing a microfluidic chip consisting of a tissue chamber in contact with a blood vessel. Such a system would allow fluids or drugs to be fed into the chip and their interactions with cells studied. They entrusted the task to Nilesh Kumar, a PhD student who had joined Prosenjit’s lab and was being jointly supervised by him and Ramray.
Nilesh was excited by the prospect of applying mechanical engineering principles to design the device. He started by surveying existing microfluidic OoC models in the market that have provisions for passing fluids through them. Most of the chips had cell layers stacked on top of each other, which made it harder to analyse under a microscope. This meant that the team could not visualise how cells interacted with each other in this artificial microenvironment. Other OoCs that had all the cells in a single planar surface used a stiffer extracellular matrix (ECM) gel because if the flow rate of the fluids changed, it could wash away cells in the matrix – this limited their versatility. Chips printed using 3D bioprinting had leakage issues.
Nilesh decided to take a different approach. To begin with, he used lithography and dry etching to fabricate a mould from a polymer called polydimethylsiloxane (PDMS). The fabricated mould has two compartments – a long vascular channel which connects perpendicularly to a circular hollow chamber. The vascular channel has an inlet and an outlet for passing fluids. The hollow chamber acts like the organ microenvironment and has a large reservoir where nutrient media is added to keep the cells alive.
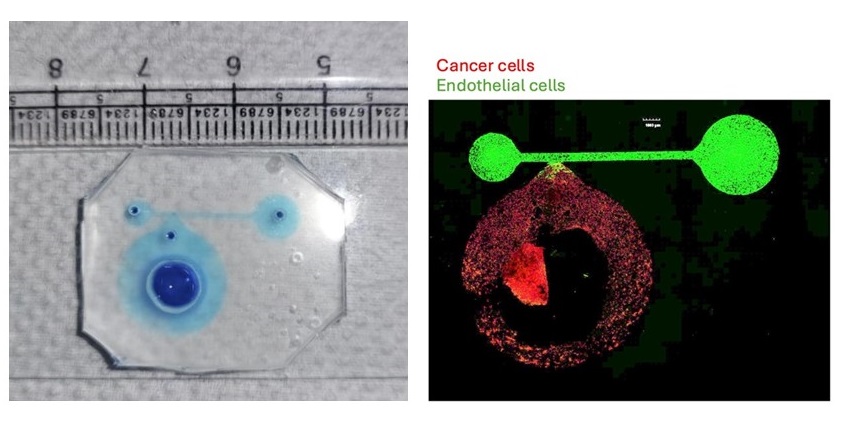
Nilesh used a low concentration of collagen to mimic breast tissue, mixed it with cancer cells, and poured the mixture into the hollow chamber. Once these cells stabilised, he then coated the vascular channel with its native extracellular matrix laminin-rich basement membrane. Next, he poured in the endothelial cells that form the inner lining of a blood vessel. Afterwards, he washed the vascular channel to remove excess cells.
But the team found that keeping the device stable was a challenge. When a fluid was passed through the device, the force of its flow would wash away the softer collagen cells that were in contact with the vascular channel. To keep the collagen cells in place, Nilesh went back and added arrays of zigzag pillars in the fabrication step. These pillars are placed near the entrance of the hollow chamber where the breast-like tissue that is formed comes into contact with the artificial blood vessel. “The circular pillars act like tree roots that grab the soil during a flood,” Nilesh explains. He also added a small opening near the pillars through which new cells could be injected without disrupting the cells in the vascular channel.
At first glance, the entire device looks transparent with a squishy PDMS layer concealing the tumour chamber and vascular channel. Small holes that are drilled into the PDMS can be seen which are the inlets for the fluids and nutrient media. The entire device rests on a glass slide which helps visualise the cells under a microscope.
Using this setup, the team was able to observe how the cancer cells interacted with endothelial cells in the blood vessel. They found that over the course of seven days, the cancer cells slowly moved out of the breast tissue into the blood vessel. The system allowed the team to look at cancer cell movement in real time. “Cancer cells are known to attract endothelial cells towards them, but to be able to see that even in such an engineered environment was pleasing,” Ramray explains.
The team has applied for a patent on their OoC model. They are hoping to use the device to test out new drug therapies for cancer cells as well as ageing stressors.
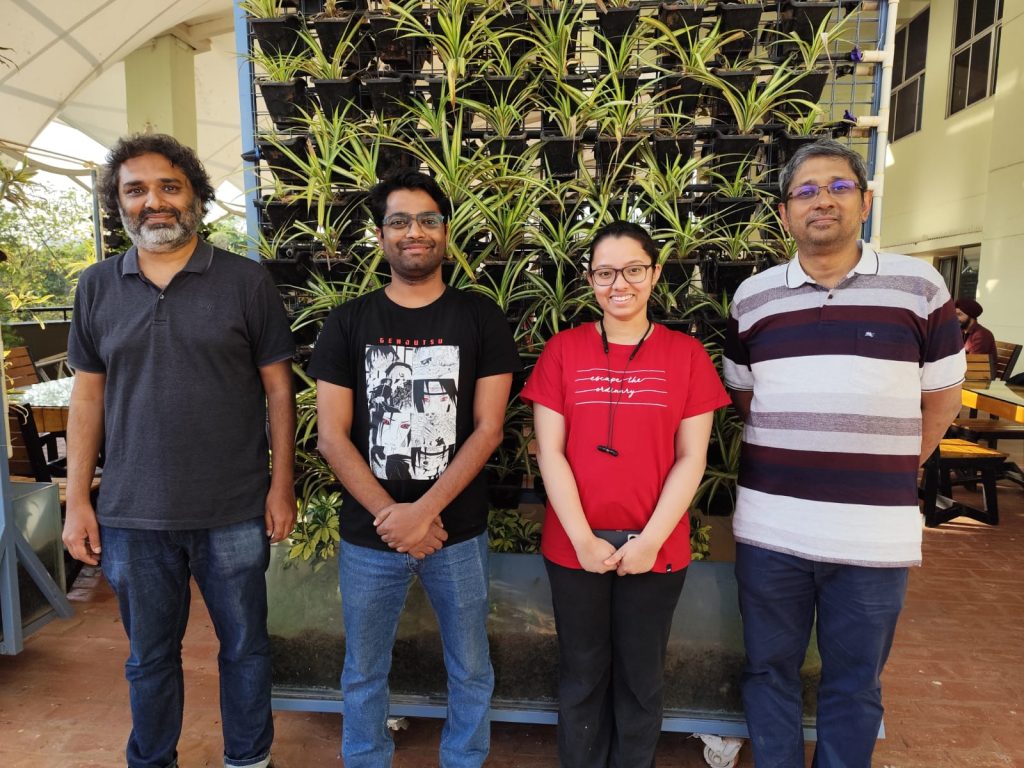
As an example, the group sought to find out whether inflammatory stressors associated with diabetes or ageing could enhance cancer cell progression. With the help of Bidita Samanta, another PhD student jointly supervised by Prosenjit and Ramray, the team selected methylglyoxal – a compound produced during glucose metabolism in greater concentration in the blood of diabetic and ageing persons – and tested its effects on cancer metastasis.
Working with Varun Raghunathan, Associate Professor at the Department of Electrical Communication Engineering (ECE) and his student Jyothsna KM, they found that methylglyoxal systematically weakened the three barriers that stood in the way of cancer cell invasion: collagen in the tissues, endothelial cells in the blood vessel, and the basement membrane matrix of the blood vessel, thus aiding cancer intravasation. Ramray says: “The surprising, insightful element was that we not only had a good device but were also able to use it to shed some new biological insights.”