3D replicas of tissues in the lab are enabling scientists to get better insight into cell organisation and behaviour
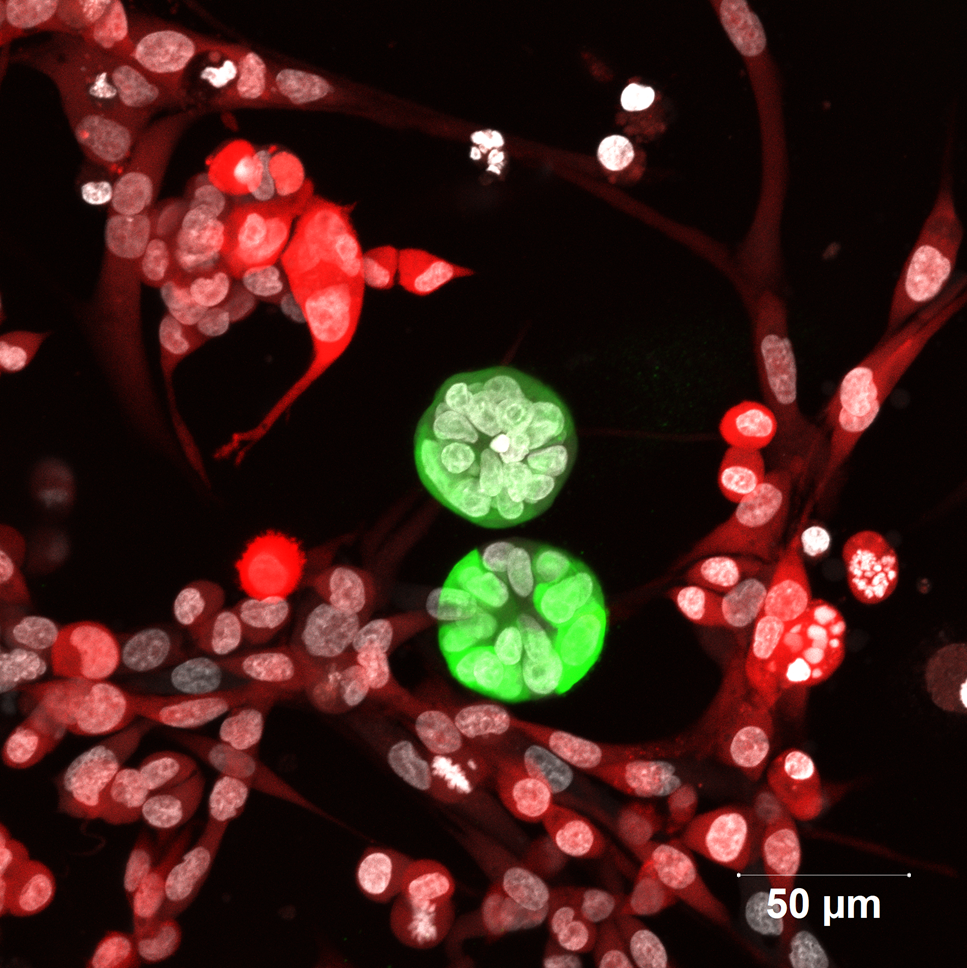
In September 2022, the US Senate unanimously passed the FDA modernisation Act 2.0 bill, which suggests reforms to the archaic Food, Drug, and Cosmetic Act that mandated testing drugs and cosmetics on animals for toxicity studies. Under the new bill, animal testing is no longer compulsory. Besides hoping to put an end to the ethical controversies surrounding animal testing, the bill encourages the development of better alternatives to animal testing as well.
But even before the bill was introduced, scientists had been working on alternative strategies to study cell and tissue behaviour in the lab without animal models. Biologists and engineers have joined forces to design systems that can recreate tissues as they are found inside the human body. However, one of the key challenges they face is that not all tissues have simple geometries. The intestinal lining, for example, is not a flat sheet – it has multiple protruding folds like a creased blanket, which increases the surface area available for food absorption.
Such complexities in tissue architecture cannot be recreated in the lab by using traditional cell culture techniques, which involve growing cells in flasks or dishes supplied with nutrient media. In these dishes, the cells attach to the surface and form a flat sheet on which biologists can perform experiments. However, what scientists see happening in these 2D cell sheets may not reflect what actually happens inside the body, because most tissues have complex 3D architectures, behaviours and properties. Cancer cells, for example, migrate very differently in a lab dish compared to how they move inside the human body. This is why many drug studies that show promising results in 2D cell cultures in the lab fail in subsequent clinical trials. And this is why researchers are rapidly moving towards organoids or 3D cell culture systems.
One example of such an organoid is a mammosphere. Mammospheres are breast tissue-derived cells arranged as dense spheres floating in a nutrient solution. Annapoorni Rangarajan, Professor at the Department of Molecular Reproduction, Development and Genetics (MRDG), uses these mammospheres to study breast cancer in her lab. To prompt the formation of these 3D structures, her team grew cells in specialised flasks in which cells cannot stick to the bottom. While most cells died due to the lack of a surface to attach to, the remaining proliferated to form floating mammospheres, enriched in stem cells.
Unlike the floating mammospheres in the lab, both cancer and healthy cells inside the human body actually attach to a substrate in the breast. Different tissues of the body have substrates with varying stiffness and protein composition. This substratum, onto which the cells latch and grow, is called the extracellular matrix (ECM). To mimic the ECM in 3D, Anu’s team collaborated with Kaushik Chatterjee, Associate Professor at the Department of Materials Engineering. In place of the substrate found in the breast tissue, they used porous PCL (Poly-Caprolactone) substrates synthesised in the lab that mimicked breast cancer stiffness. However, they found that the patient-derived cells could not latch onto and survive for long on the PCL scaffold on their own. So, they first cultured fibroblasts – cells which secrete ECM components such as proteins – on the PCL scaffold. After the fibroblasts had secreted ECM components on the PCL scaffold, they were killed. Then, breast cancer cells from human patient samples could attach to the scaffold and form 3D organoids, similar to how tissues are arranged inside the body.
Organoids are also the model system used to study cancer in the lab of Ramray Bhat, Associate Professor at MRDG. To study ovarian cancer, the group uses spheroids – cell clusters found in ovarian cancer patients. They grow spheroids in combination with cells of the gut lining to study how cancer cells colonise and penetrate the tissue to reach other organs. They also grow 3D cultures of breast cancer cells embedded in substrates containing fibronectin, collagen, hyaluronic acid and matrigel – important components of the breast ECM.
Besides the ECM, the tissues in our body are also in contact with bodily fluids and other cell types. The local environment around a tissue is called the tissue microenvironment (TME). The group mimics the TME found in diseased conditions by altering the composition of the fluid in which cells are cultured in the lab. “These are complex cell systems where we can look at the intersection of cancer and non-communicable chronic diseases such as diabetes,” explains Ramray.
While most cells in tissues are attached to the ECM, some cells, such as red blood cells and white blood cells, flow with blood in blood vessels. Blood vessels are also the highway for cancer cells to migrate from one organ to another, a process called metastasis. During metastasis, some cancer cells may also be found flowing inside the blood vessels. To isolate cancer cells from blood vessels, Ramray’s group collaborated with Prosenjit Sen, Associate Professor at the Centre for Nano Science and Engineering (CeNSE). Using microfluidics, they have devised narrow tubes that mimic blood vessels and by tweaking the tube size, are able to replicate how blood and cancer cells flow inside our bodies.
To enter blood vessels from the primary tumour site, cancer cells usually bore their way through the ECM of the tissue. An alternative route weaves through free spaces in the ECM, called interstitial spaces. “Cells of sizes of a few 100 micrometres can squeeze through channels of about ten micrometres, which changes the mechanical properties of cells. We try to understand whether we can use this change in mechanical properties to kill cancer cells using external mechanical forces without damaging normal cells,” explains Ajay Tijore, Assistant Professor at the Centre for BioSystems Science and Engineering (BSSE). To mimic the journey of cancer cells via narrow interstitial spaces, the group uses a combination of microfabrication and soft lithography techniques to construct artificial microchannels. Cancer cells are passed through these channels and are subjected to ultrasound-generated mechanical forces to see if they can be killed.
Ajay’s lab is also working on mimicking the ECM at the nanometer scale. Cells use a transmembrane protein called integrin to attach to the ECM. One end of the protein lies inside the cell, and the other end latches onto specific proteins at the ECM which repeat at regular intervals. To mimic these proteins, the researchers use gold nanoparticles to which integrins bind. These nanoparticles also control the spacing between consecutive integrin molecules – an important property that differs in normal and cancer cells – providing a system to study how receptors contribute to mechanical weaknesses in cancer cells.
“What we have done until now has been static 3D organoids. Moving forward, we would like to bring together multiple organs at homeostasis on a single chip,” says Ramray. Such efforts tie into the emerging personalised medicine revolution: soon, scientists may be able to tailor treatments and drugs to suit each individual by replicating entire human organs or organ systems – a mini “you” in the lab.