Kausik Majumdar’s lab taps into atomically smooth interfaces for developing devices to realise the promise of quantum technologies
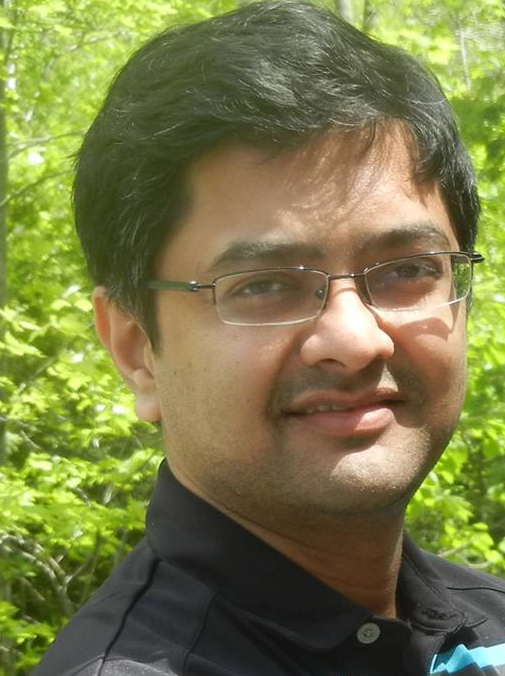
Even before pursuing his bachelor’s degree in Electronics and Communication Engineering, Kausik Majumdar knew he had a penchant for research. But his path to becoming a faculty member weaved through both industry and academia.
After completing a master’s degree from IIT Delhi in 2005, Kausik went on to work in the electronic design and automation industry, followed by a PhD from the Department of Electrical Communication Engineering (ECE), IISc. After his PhD, Kausik joined a device research consortium called SEMATECH in Albany, USA, as a research engineer. What set this place apart, he recalls, is the fact that big names in tech such as IBM, INTEL, and others would come together to work on futuristic devices. Their efforts were 10-20 years ahead of their industry counterparts, he adds. “This gave me a lot of exposure towards industrial as well as academic research.” Armed with this experience, Kausik joined IISc as an Assistant Professor in 2015. Today, his Quantum Electronics lab works on developing hardware components to harness the power that lies in the behaviour of subatomic particles in the quantum realm.
Quantum technologies are expected to perform much better than their classical counterparts in many applications. One of their building blocks is single photon emitters (a photon is a packet containing a tiny amount of light-energy). In quantum computing, for instance, unlike classical computing where electric pulses are used to relay information, the photon represents the smallest unit of information transfer and processing. Quantum communication uses the polarised states of photons to encode information for achieving highly secure communication. Similarly, some quantum sensing technologies also rely on single photon generation. The use of photons holds the promise of a tremendous improvement in capability and performance of quantum technologies over their predecessors.
Kausik’s lab is working towards achieving a fast, on-demand generator that can produce photons for such applications. They have progressed to preliminary demonstrations of single photon emission. They have also developed a highly efficient detector that can pick up individual photons at a wavelength (1550 nm) that is crucial for quantum communication and sensing. The detector works at room temperature, a significant advantage over other techniques that often require cryogenic cooling, which drives up their cost and restricts their applications.
Another device from the lab that made headlines recently was a True Random Number Generator (TRNG). Random number generation sits at the heart of secure data encryption. “Random number generation is ubiquitous nowadays,” remarks Kausik. It has applications ranging from securing communication and banking transactions to academic efforts like developing large-scale biological simulations. Even dealing cards in casinos, for example, requires such randomness.
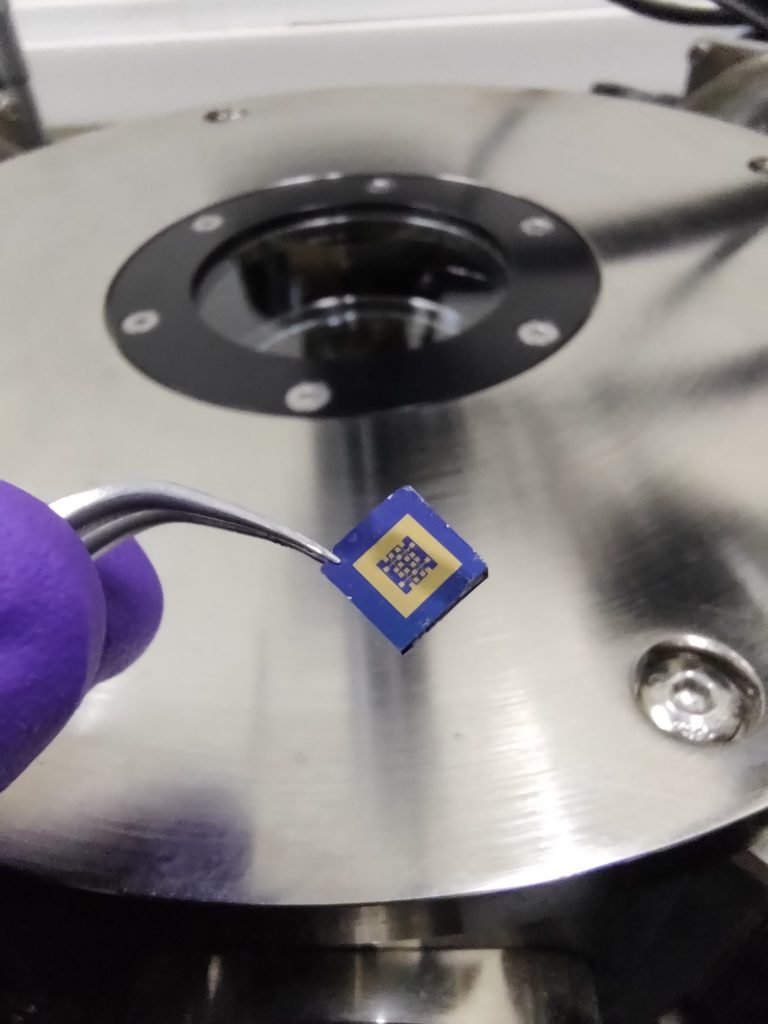
A random number generator can help create unique codes that can keep communications secret from any and all unintended recipients or prying third parties. A string of numbers generated by computer programs and some marvellous mathematical operations lock the information in such a way that it can only be read by the intended recipient, who uses another set of randomly generated numbers to unlock the information. For this communication to be secure, the numbers have to be random to keep unintended users from predicting them and unlocking the information.
Conventional computer programs that generate these numbers aren’t truly random because they take the help of certain pre-arranged objects like inbuilt mathematical formulae or programmed tables. These are hence known as pseudo random number generators. Instead, Kausik and his team developed and demonstrated the working of a true random number generator which harnesses the power of randomness inherent at the quantum level. This randomness is the motion of electrons, which is completely unpredictable.
By fashioning a device out of atomically thin layers of materials like black phosphorus and graphene to trap these moving electrons, which would tell them when the materials did so by changing the resistance of the device, the team successfully managed to harness the randomness inherent in the motion of electrons and use this to generate true random numbers for securing private data. Their device turned out to be a record-breaker: its min entropy – a parameter used to measure the performance of TRNGs – was found to be 0.98, the highest reported value so far in the world, according to a standard set by the USA’s National Institute of Standards and Technology (NIST). Kausik and his team plan to improve the data handling capabilities of the device without compromising the quality of randomness so that the device can be used for applications like quantum communication.
Yet another area of interest for Kausik’s lab is studying the unique characteristics of semiconductor materials at the quantum level. They do this to look for ways to push the boundaries of device capabilities. One such area of research is tunnel diodes – semiconductor materials in which, contrary to the norm, current decreases as voltage increases. The beauty of this property lies in the fact that the current passing through the device generates power instead of losing it when flowing through a conventional resistor, earning this phenomenon the name negative resistance. Scientists have, therefore, been keen on studying and improving the performance of such tunnel diodes.
Kausik’s lab has used a special class of material systems made up of very thin layers of certain compounds, which makes it possible to manipulate even individual electrons at atomic junctions. He explains, “Using layered materials to make tunnel diodes, we have achieved the cleanest [voltage-current] characteristics in a device.” The clean characteristics, he explains, is the tightness of the relationship between voltage and current. His lab’s efforts have surpassed previous attempts by other labs to achieve such high performance, he adds.
Kausik’s lab is also excited about excitons – quasi-particles within semiconductors made of a negatively charged electron and a positively charged empty space called the hole, which underpin some of the inherent properties of semiconductors. The team has studied the force of attraction between the electrons and holes, and how they combine to generate light. This study led to an important breakthrough of achieving 100% polarised light – the electric field of light oriented towards one direction only – from the interaction between the electrons and holes. The emission properties achieved is an important milestone in excitonic emission research.
With the quantum revolution rapidly taking over the world, Kausik’s lab is keen on pushing forward with developing hardware components that can quickly be deployed in emerging technologies. Because some of the devices he is developing can have diverse real-world applications, Kausik is also eager to commercialise these devices, working with industry partners.
Although research consumes a large part of Kausik’s daily routine, when things do not seem to be going right, he likes to step away from the lab and immerse himself in a good 90-minute game of football. While coming back from the field, he says, his mind is ready to churn out new ideas that can be tested out in the lab.
Drawing insights from his own journey as a scientist so far, Kausik has this piece of advice for aspiring researchers: “Think deeper than you think you are capable of and have the right motivation. I think the person who can motivate you the most is yourself.”
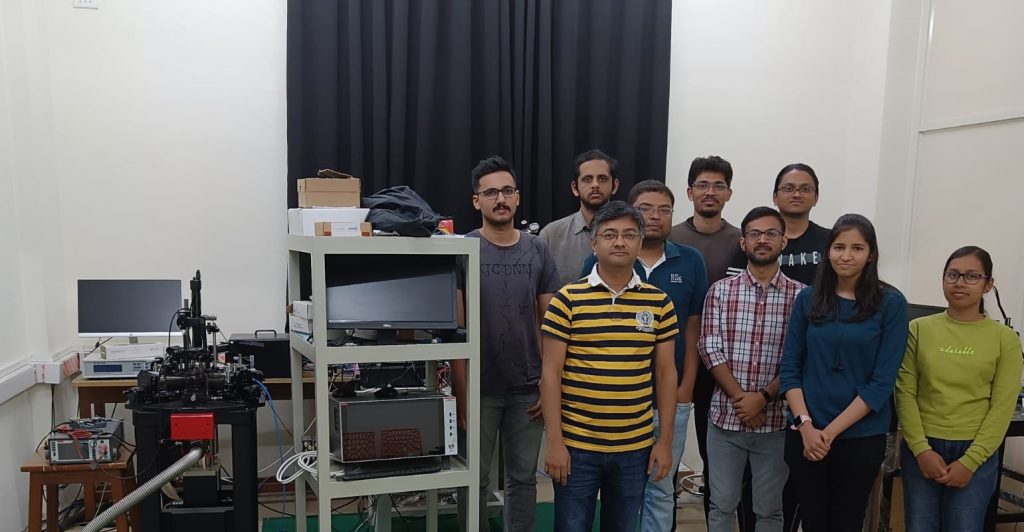