By designing gas sensors that incorporate nanomaterials, IISc researchers are developing solutions to problems in many fields, including space research
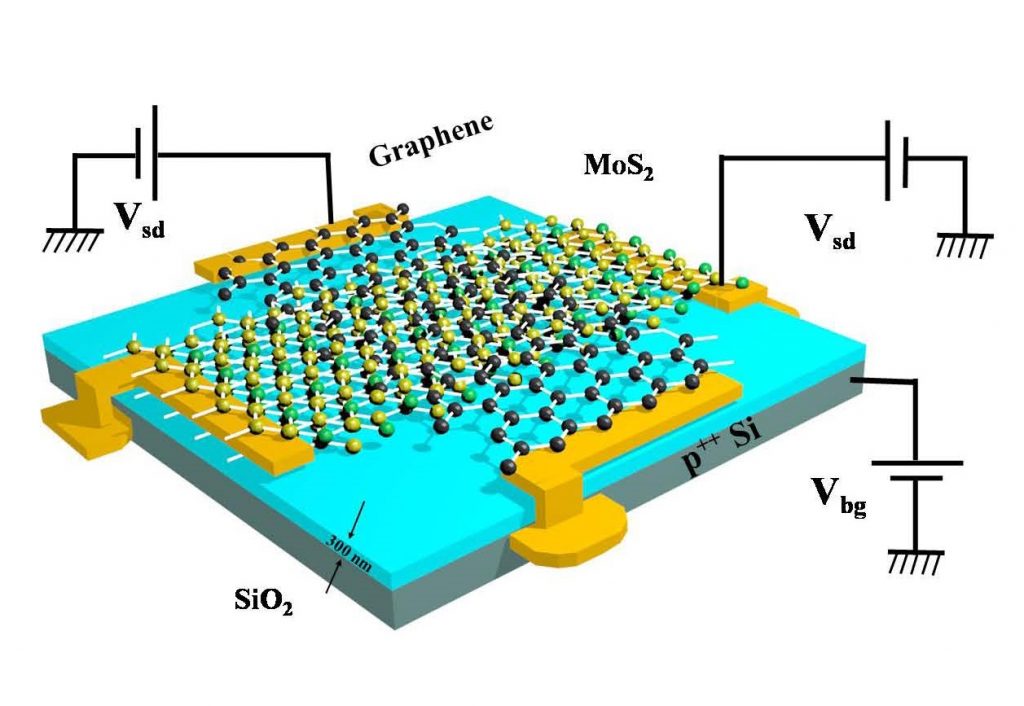
“Our eventual goal is to construct an electronic nose,” reveals Navakanta Bhat, Professor, Centre for Nano Science and Engineering (CeNSE). He is referring to a yet-to-be developed electronic device whose sensors would be able to detect as many odours as a human nose. Not long ago, such a device would have been in the realm of science fiction. However, with the advent of nanoscience – the field that is currently revolutionising everything from sunscreen to computers to tennis equipment – electronic noses could soon become a reality.
Though such an electronic nose is yet to see the light of the day, nanoscience is dramatically altering the field of gas-sensing. For instance, nanomaterials-based gas sensors are becoming essential in monitoring toxic gases in the atmosphere as we attempt to combat air pollution. Additionally, gas sensors can now be used to find hidden explosives by sniffing out trace gases that form a part of their chemical signature. They are also finding applications in chemical industries which work with gases such as compressed natural gas, carbon monoxide and nitric oxide, to ensure that there is no leakage from pipelines. Hydrogen sensors are now commonly used in space research to detect leakage as hydrogen is used as a fuel in rockets. Another potential application of gas sensors that is only just starting to capture the imagination of nanoscientists is in healthcare. With every breath, we let out thousands of organic molecules. If researchers can develop devices that measure the concentration of molecules associated with certain diseases, they could help doctors diagnose and determine the extent of these diseases.
A nanomaterial is any substance that has at least one dimension in the nanoscale – which is a thousand times smaller than the microscopic scale and just about one billionth of a metre. S Venugopal, Associate Professor at the Department of Chemical Engineering, explains why the application of such materials is game-changing: “For gas-sensing applications, the use of metallic nanomaterials is mainly driven by their large surface area. Whenever you want to sense a gas, the molecules of the vapour need to interact with the solid or liquid phase of the sensor, and the extent of the interaction is determined by the interfacial area. As nanoparticles give us a very large interfacial area, they are ideal candidates for gas-sensing.”
Venugopal’s lab, in association with ISRO, is developing hydrogen gas sensors using metallic nanomaterials such as palladium. In the nanoscale range, palladium particles selectively absorb hydrogen gas to form palladium hydride, which causes them to swell up. This increase in volume makes them forge new electrical connections with neighbouring particles, resulting in a decrease in resistance. The change in resistance can be detected by a corresponding increase in electric current. His lab is currently optimising these sensors so that they work at room temperature, and also developing processes to manufacture these sensors on a large scale.
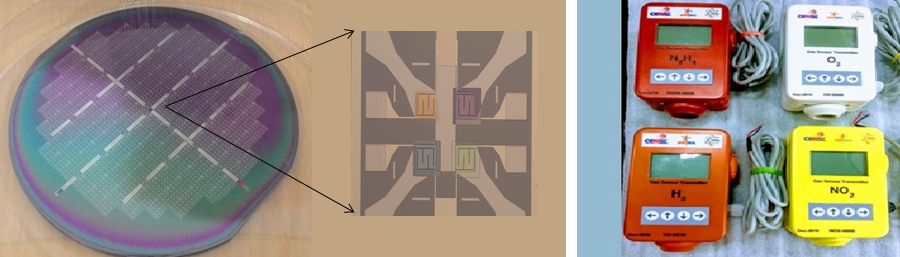
Navakanta’s lab, on the other hand, is designing gas sensors that work on a chemiresistive principle – exploiting chemical reactions in nanomaterials to detect a change in resistance and thereby the type or quantity of gas. Some semiconductor nanostructures, such as metal oxides, undergo redox reactions when they encounter the target gas, which changes the resistance of the device. “Each metal oxide has an affinity for a particular gas, which allows it to selectively detect that gas,” he says. By manipulating this property of metal oxides, his team has successfully constructed sensor arrays that incorporate several sensors on a single device, which can be used to detect a variety of gases in the environment. Presently, his team is working on making the sensor array even smaller. “The intent is to make these arrays smaller and smaller because, in the nanoscale range, the power consumption decreases, the sensitivity increases and the response time of the sensors is faster,” he explains.
To miniaturise the sensor array, Navakanta’s team synthesises nanotubes – structures that consist of metal nanowires (the core) encapsulated inside metal oxide rods (the shell), which are then connected to circuits for gas detection. In such structures, the properties of the sensor can be regulated by changing the core-to-shell thickness, which increases their sensitivity. Other than metal oxides, his lab also uses a certain class of compounds called transition metal chalcogenides, to design extremely thin nanosheets which display enhanced sensitivity and can work even at room temperatures (redox reactions generally occur at high temperatures in traditional sensors). These nanosheets are more efficient because the reactions do not require a heater which is typically used in such devices.
Another group led by Abha Misra, Associate Professor at the Department of Instrumentation and Applied Physics, is working on a new development in gas sensing. They are designing sensors as memory devices that “remember” their interaction with a particular gas and retain it as a molecular memory. “Gases are generally detected by virtue of electrons or charges that they exchange with the sensing material. If these charges can be trapped and retained, then a molecular memory can be created for a number of gases each time the device is reset,” spells out Abha.
In these devices, a single sensor can detect a number of gases as the process does not depend on the material, but rather on the modulation and operation of the sensor. These sensors consist of a nanofilm (a few layers) of molybdenum disulphide, a semiconductor, along with a layer of graphene. Both of these layers are connected to two independent electrical sources, so that the external electrical field of the sensor can be suitably fine-tuned, thereby attracting a specific target gas. Apart from quantifying and detecting gases, these sensors can also be used as molecular switches for devices, and even as non-volatile memory devices to store data about gases.
While new applications for nanomaterial-based gas sensors are being found thick and fast, the ultimate goal of the field remains the development of an electronic nose, Navakanta points out. But building an electronic device that can mimic the human nose is a tall order because the human nose has an equivalent of about six million sensors and can detect over a trillion smells. However, the advent of Big Data and AI could be a big step forward in making such a device come true, he feels. “Once you have a million signals coming from a sensor it also becomes a data science problem,” he says. “My PhD students are just beginning to explore the use of AI in sensor arrays.”