Prerna Sharma’s research focuses on understanding the nature of soft matter at the interface of biology and physics
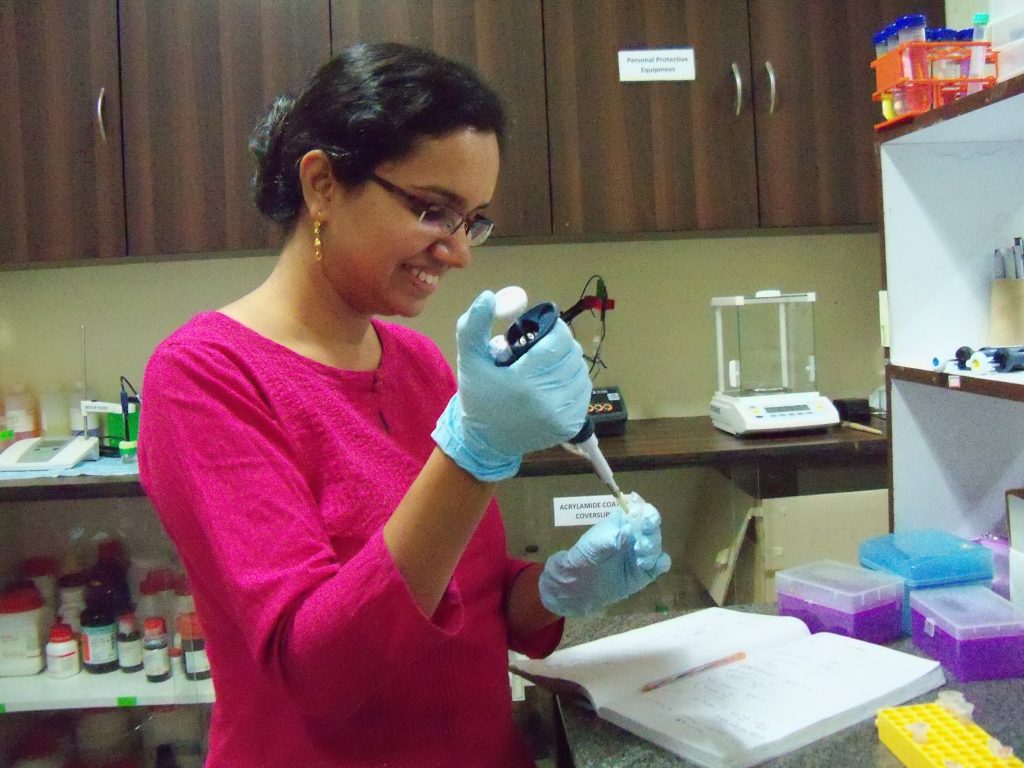
Membranes and colloids aren’t exactly the first things that come to mind when one thinks of physics. But it took listening to just one talk during her BSc at St Stephen’s College for Prerna Sharma to know that is where her destiny lay. Her eyes light up as she recalls that moment. “I knew this is what I wanted to do!” She didn’t dip her toes into biophysical waters immediately, though. Her PhD work was broadly oriented around the fluid dynamics of colloids, mixtures in which one component is finely dispersed within another. These mixtures can be biological, such as milk, which is a colloid of fat and water, or non-biological, like fog, a colloid of water crystals and air.
By the time she started her post-doctoral research at Brandeis University in the USA, she had developed a series of tools for studying simple colloidal systems which she wanted to apply to more complicated (read: biological) cases. But unlike conventional biophysicists who study things at the molecular level, like protein folding and protein-protein interactions, her interest was in larger structures.
Now an Associate Professor in the Department of Physics, Prerna focuses on soft-matter physics, the study of systems that are ‘squishy’ or easily deformed. One of the major systems they study are colloidal membranes. Specifically, they focus on rod-shaped viruses and self-assembly. “Imagine putting a bunch of parts in a box and shaking it, resulting in a fully-assembled computer,” says Prerna. That is essentially what her lab does with these viruses. When added to a liquid, under the right conditions, the viruses assemble into a single-layered sheet and orient themselves in the same direction, forming what is called a colloidal membrane. Their lab looks at how these viruses assemble spontaneously under different physical and chemical conditions at the micron scale.
The shapes of colloidal membranes can change depending on how they interact with the components of their environment. One of the ways in which Prerna’s lab examines these membranes is by crystallising them, which causes the positions of individual viruses to become fixed and the membrane to adopt a certain shape. In one study, they found that after crystallisation, these membranes became unexpectedly wrinkled and buckled, due to a property called “chirality”.
A typical example of chirality is the human hand. The mirror image of a left hand is a right hand, not another left hand. Similarly, the viruses exist as ‘left’ and ‘right’ versions. Prerna and her team found that while most of the membrane crystallised, the edges remained fluid-like. The viruses in the fluid regions were able to move and shift positions in response to the forces exerted due to chirality. However, they were unable to do so in the solid, crystallised part, leading to wrinkling and buckling. “It was an unexpected discovery,” recalls Prerna, smiling. It was the first major finding from her lab, published in Nature Communications.
Since then, the lab has also made significant progress in understanding the nature of the movement of cilia, which are tiny hair-like structures on cells (singular: cilium). Cilia are “active” filaments that perform important functions within the cell, and across tissues. Prerna and her lab purify cilia from an alga called Chlamydomonas and study their ‘beating’ movement in water. The reason they are interested in cilia is because these rod-shaped structures can propel themselves forward using an internal mechanism, without needing an external force.
As the cilium moves by ‘beating’ or oscillating in a fluid, the stresses generated inside its body need to be balanced out, ideally by external friction – the drag exerted by the fluid on the cilium. However, Prerna’s team realised that the effect of this external friction was too small. Instead, rearrangements within its structure itself were creating enough ‘internal friction’ to help the cilium move, the team found. These experiments were the first to unambiguously show how internal friction drives cilia movement.
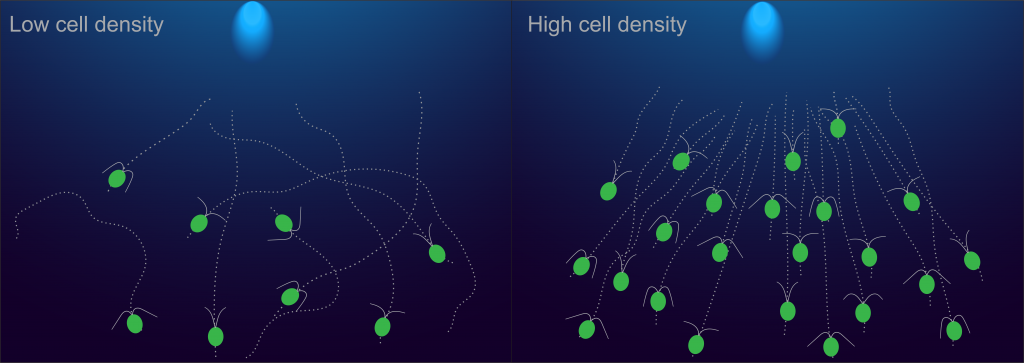
A third area in which Prerna’s lab works is collective phototaxis, the spontaneous movement of certain single-celled organisms towards a source of light. Large groups of Chlamydomonas are known to be faster than a single cell at detecting light and orienting themselves towards it. Her lab showed that the reason behind this is the crowding from being in a large group, which causes their normal rotating and tumbling motions to slow down, leading to greater efficiency in detecting light. This finding received a lot of attention on Twitter, and one person even stated, “How to do better? Slow down!” while citing her research.
Prerna’s work has not only the potential to advance our knowledge of self-assembly in biological systems but also industrial and medical applications. Understanding how thin sheets behave, for example, and pushing them into well-defined shapes can lead to better manufacturing processes. Since the beating of cilia is also responsible for cleaning our lungs of any debris that finds its way there, the lab’s work could also one day lead to the creation of a personalised medicine platform for human lungs, according to Prerna.
“I’m not planning the personalised medicine platform immediately,” she laughs. In the short term, she wants to create and work with colloidal matchsticks, which are long viral rods with a small bit of protein at one end. These matchsticks naturally assemble into membranous sheets just like the viral rods. Such objects fascinate both biologists – who seek to study how they interact with other biological molecules – and physicists – who might gain insights into symmetry and self-assembly from studying them – alike. For example, they could serve as a platform to study how drugs interact with the protein carried by the matchstick.
In the long term, Prerna wishes to explore deeper questions about emergent behaviour – new behaviours that arise when similar objects come together to form a complex system. “Now that I know how a single cilium behaves, I would like to put two of them together and see if I can predict their behaviour,” she states. “And once I know that I would like to look at the behaviour of a larger number. Maybe a whole carpet of cilia.”
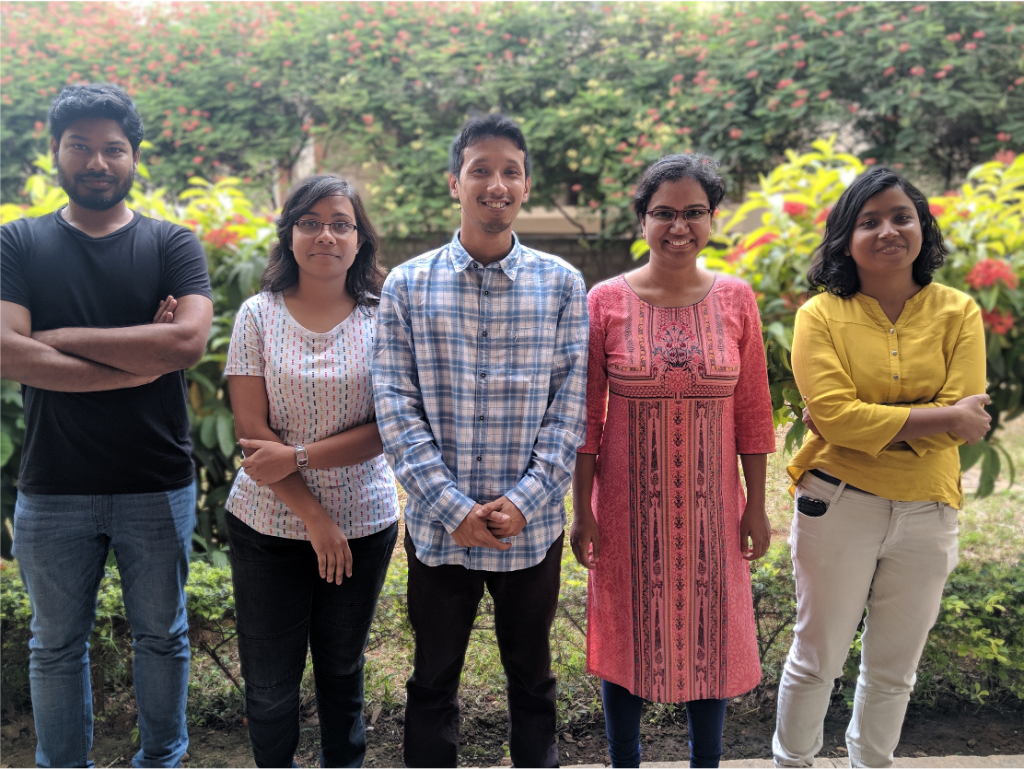