The exciting field of active matter seeks to uncover complexities of collective behaviour
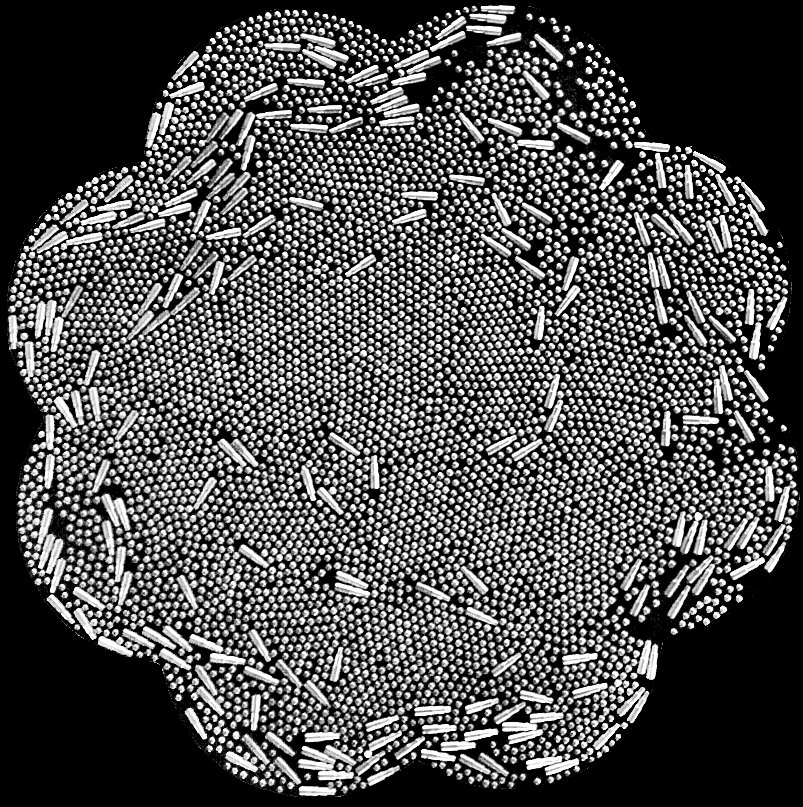
How do flocks of birds and schools of fish move together in beautiful patterns? What do they have in common with a crowded Mumbai railway station? The answers to such questions lie in the field of active matter.
Active matter refers to a collection of particles or entities with the ability to convert energy to useful work. “Human beings dancing in a concert is active matter,” chuckles Prerna Sharma, Associate Professor at the Department of Physics. Active matter can exist at different scales. “The living tissue of which an organism is made is active matter, made up of cells that are the active agents. In bird flocks, the organisms themselves are active agents and the flock is the active matter,” explains Sriram Ramaswamy, Honorary Professor at the Department of Physics, and a pioneer in the field.
Using principles of active matter, physicists at IISc are trying to discern the general rules and principles governing the organisation of biological systems: from swarms of bacteria to flocks of birds. Biologists at IISc are also studying active matter to understand phenomena like cancer spread and sperm motility. Engineers are using active matter to get a better understanding of industrial and clinical problems, like flows in microchannels.
Missing equations
The beginnings of the field of active matter can be traced back to physicists studying soft matter about 30 years ago. Anything that is soft and can be easily deformed falls under soft matter. Soft matter shows interesting properties like viscoelasticity – the ability to flow like a fluid but also retain shape after deformation like a solid. Physicists were interested in soft matter because it arises in living systems. Life itself, and many phenomena in the living world (such as the collective movement of cells to form different tissues during embryo development) are examples of emergence – properties of the collective that one can’t anticipate from the behaviour of individual particles. Physicists studied, in theory and experiments, the collective emergent properties of living systems, and tried to write equations for a collection of particles that can consume energy to propel themselves and move. Thus was born the field of active matter.
A major breakthrough came in the year 1995 when John Toner and Yuhai Tu, condensed matter physicists then at IBM’s Watson Research Center, wrote equations to describe active agents such as birds moving as a cohesive flock, in terms of the local density and velocity fields, not the individual creatures. However, the equations did not take into account the hydrodynamic interactions between the agents within a fluid medium via flows created by their swimming. Sriram’s group came up with equations that included such missing fluid interactions. Their equations predicted an unexpected outcome: that the orderly arrangement such as those seen in bird flocks is drastically disrupted by the viscous hydrodynamic interaction.
Slow is smart
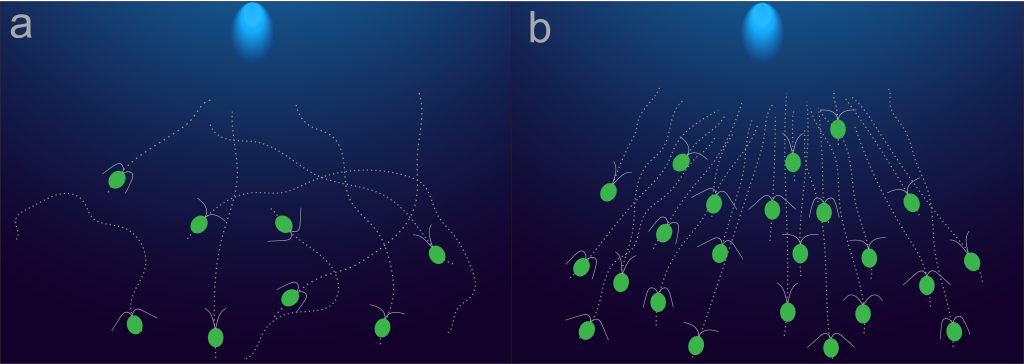
Prerna, a physicist by training, also uses the framework of active matter to study biological systems. Her group studies how Chlamydomonas reinhardtii, single-celled algae, move towards light. While biologists have been studying this “phototactic” nature of algae in individual cells, Prerna’s lab wondered how cell numbers might affect their movement towards light. “If you observe a collection of cells running towards light, you will see that a certain fraction is running in the wrong direction,” says Prerna.
The percentage of cells running in the wrong direction increases with increasing density, up to a certain threshold cell density. After the threshold density however, a higher fraction of cells move correctly towards the light source. “That is, cells tend to do better when there are a lot more of them together,” says Prerna. They speculated if quorum sensing – collective sensing via chemicals in bigger groups – might be the reason, but they could not find any chemical mechanism to explain their results. “Instead, what we found is that the speed of movement of the cells decreases when you increase the concentration and that decrease in the speed is what makes them perform better. That decrease in speed then feeds back into how these cells detect light and enables them to make fewer mistakes,” explains Prerna.
Out in the real world
“Just like we study states of matter like solids, liquids, or maybe magnets and plasma, we study this [active matter] as a branch of physics,” says Sriram. He cautions, however, that not all predictions based on their equations would have real world applications.
But some engineers think that such findings can provide solutions to industrial and clinical problems.
Trained as an engineer, Danny Raj, a DST-Inspire faculty member at the Department of Chemical Engineering, stumbled upon the field of active matter while studying the dynamics of oil droplets in water within microchannels during his PhD. Engineers typically use the Navier Stokes equation, but it was computationally difficult to use it to design these systems. “I started with the goal of finding simpler models and hit upon the term ‘collective dynamics’, which is part of what active matter researchers are also interested in,” says Danny.
Engineers are also interested in understanding how an individual entity within a collective behaves. For instance, although birds move collectively as a flock, how does a single bird decide its direction? Individual animals tend to stick to the group to avoid getting lost and keeping safe from predators, while at the same time avoiding banging into each other.
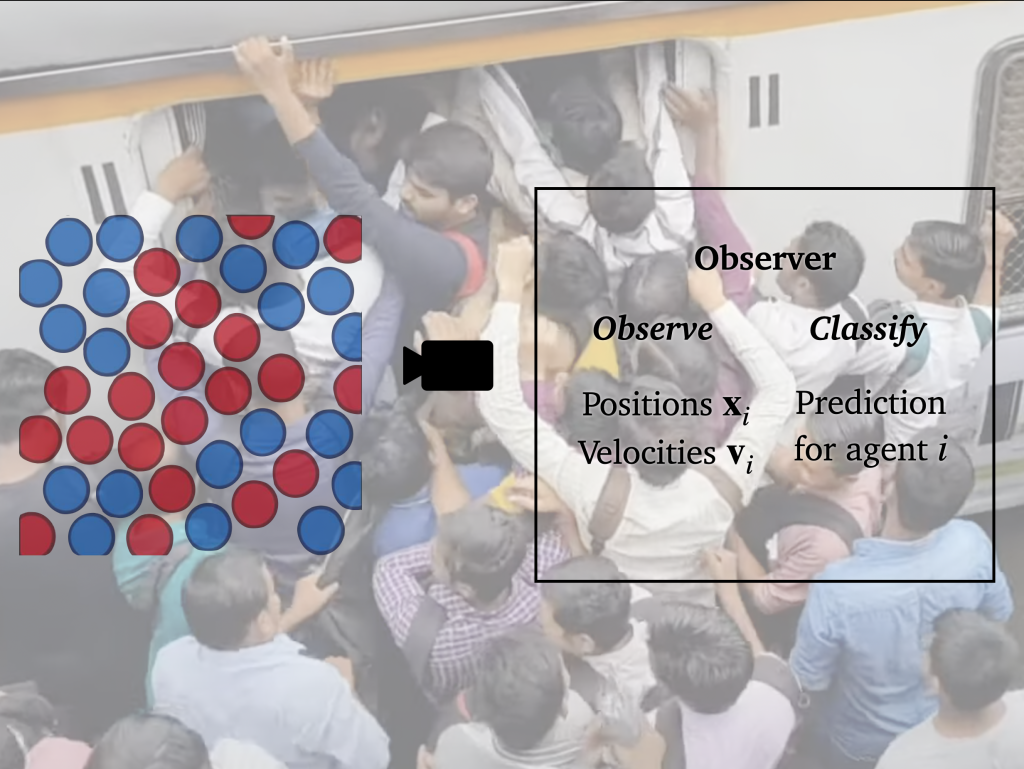
Danny’s group is working on understanding such individual behaviour. His lab uses computational tools to fit the collective data and infer individual-level interactions. “If you look at people moving in a Mumbai railway station from the top, we would not be able to find which way they want to move because they are pushed in all directions. If we looked at only one person and they move to the right, we might think that they want to move right. They might have wanted to move left but was pushed by the crowd to the right,” explains Danny. To avoid such misinterpretations of the data, it is necessary to explicitly include local-level interactions while analysing the data.
Engineers like Danny are also interested in understanding how active matter can adapt to changes in the environment. Recently, a team of engineers in Switzerland built a microrobot collective in the shape of small disks driven by a magnetic field. It can show different ‘behaviours’ such as moving through channels and passing around obstacles in air-water interfaces. Unlike conventional robots, such microrobots can reconfigure themselves and choose a specific behaviour as the environment changes, making them suitable for complex environments such as oil spills that conventional robots might not be able to navigate. By understanding active matter better, it might soon be possible to engineer such synthetic systems which show behaviours just as fascinating as living systems.