Physicists at IISc are trying to answer fundamental questions about the nature of a mysterious form of matter in the universe
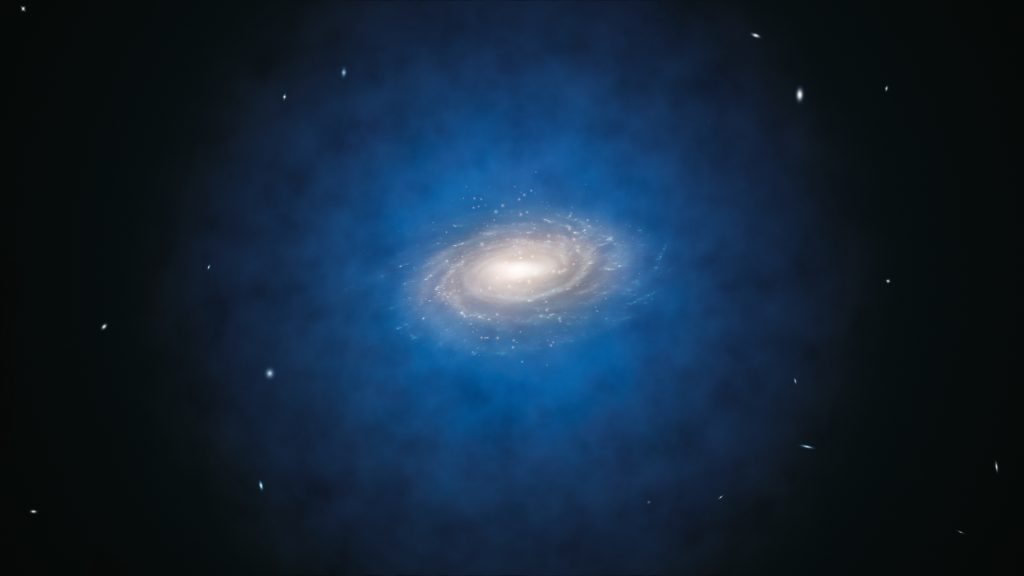
From once believing the Earth to be the centre of the universe to realising that our planet is just “a mote of dust,” as famous astrophysicist Carl Sagan put it, humans have come a long way. In the early 20th century, physicists made yet another startling discovery – all the planets, stars, and galaxies make up only about 4% of our universe. Most matter is, in fact, invisible to us – dubbed “dark matter” (DM). DM is estimated to make up around 23% of the universe, while the rest (~73%) is believed to be made up of an even more mysterious component called Dark Energy.
The earliest evidence for the existence of DM came in the 1930s. Swiss astronomer Fritz Zwicky noticed that the galaxies in the Coma cluster (about 322 million lightyears from Earth) were moving too fast to only be under the gravitational influence of the visible matter in the cluster. The vast majority of the mass of the Coma cluster seemed to be “missing.” Zwicky’s missing mass is what we identify as DM today.
The big question that physicists are attempting to answer is: What is dark matter made of?
“Since it does not radiate any light, most methods of studying dark matter rely only on its gravitational influence on visible matter,” explains Sudhir Vempati, Professor at the Centre for High Energy Physics (CHEP), IISc. Sudhir, along with Rajeev Jain, Assistant Professor at the Department of Physics has been investigating the possibility of bounding the allowed mass of DM constituents and their interactions with visible matter by studying their effect on Neutron Stars (NS).
NS are extremely dense objects that form when a star stops burning and collapses onto itself under its own gravity. Theoretically, very old NS (older than a billion years) should cool down substantially (to a few 100 K) and be impossible to see through the current telescopes. But if dark matter is present, its particles could bump against the NS or undergo a process called pair annihilation near the NS, emitting high-energy photons. Both these processes would heat up the NS and make it potentially detectable. “While this idea was around for a while, no one had quantified the actual rise in NS temperature due to DM heating while taking into account all the variables and experimental data,” says Sudhir. Remarkably, the researchers were able to calculate that the NS temperature can rise up to 2600 K in the presence of DM.
Scientists have also attempted to identify possible candidates that constitute dark matter. One of these is called Weakly-Interacting Massive Particles (WIMPs). Experiments have shown that DM density in the early universe became fixed to a constant termed the relic density, possibly due to a widely believed theory called “freeze-out”. The freeze-out theory postulates that in the early universe, there was a balance between processes converting DM to visible matter and vice versa. At some point, however, when the universe’s expansion rate became fast enough, the DM density reduced to a point where the reaction to convert DM into visible matter completely “froze out.” The relic density we observe today is the value of the DM density at this freeze-out point. Since the freeze-out mechanism requires DM-to-visible matter conversion, it necessitates that DM particles should interact with visible matter via forces other than gravity. One possibility is that they interact via the so-called “weak interaction,” a type of force that is significant only within sub-nuclear distances but becomes extremely weak at long distances. Such potential DM constituents have been called WIMPs.
Although the idea that DM may be made of WIMPs is popular, it has not been experimentally demonstrated yet. Scientists, including Sudhir and Ranjan Laha, Assistant Professor at CHEP, believe that DM might contain a different type of constituent called Feebly-Interacting Massive particles (FIMPs). They investigate an alternate proposal called “freeze-in” to explain the relic density of DM. The freeze-in mechanism postulates that there was negligible DM in the early universe. All DM was produced by the decays and annihilations of visible particles. This continued till the expansion of the universe caused the density of visible particles to become too small for DM production to continue. The freeze-in mechanism requires the interaction strength of DM particles to be much smaller than that of WIMPs (a million times smaller or less), leading to the name FIMPs for the new DM candidate.
Sudhir and Ranjan, along with Shiuli Chatterjee, a former PhD student at CHEP, have proposed that FIMPs could consist of three different particles instead of just one. “We were led to this idea by realising that the interaction strength required for FIMPs is very close to that with which electrons interact with another particle called the Higgs boson,” says Sudhir. Electron, muon and tau are subatomic particles that belong to the same family and are identical, except that they interact with the Higgs boson with different strengths and this leads them to have different masses. We do not see tau and muon easily as they are more massive and decay into electrons (the lightest of the three) very quickly. The researchers propose that FIMPs may also exist as a family of three particles just like the electron, muon and the tau (but with no electric charge) and interact only with the Higgs boson. The lightest of the three could be a stable DM candidate.
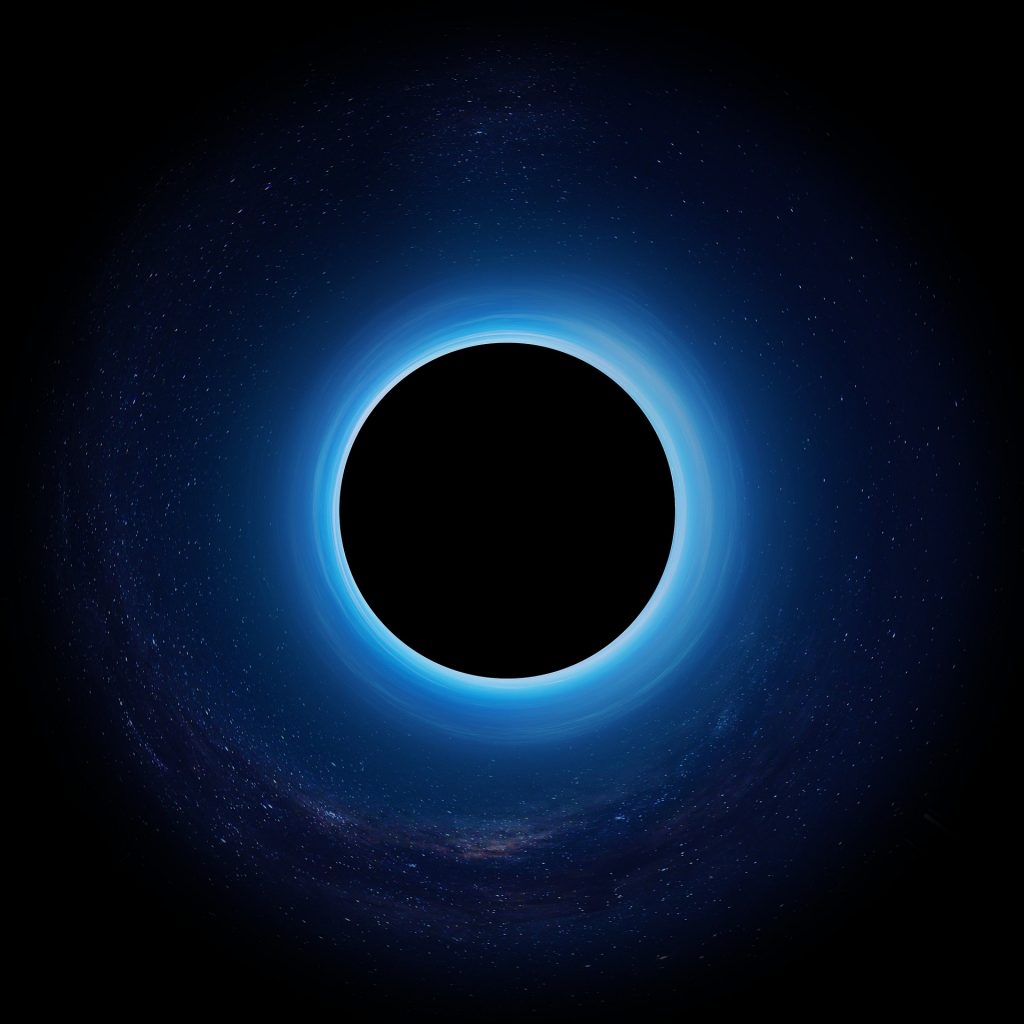
All of DM need not be composed of subatomic particles like WIMPs and FIMPs. Ranjan has been exploring the possibility of DM being composed of primordial black holes (PBHs). PBHs are hypothetical black holes that are much smaller than regular black holes and are thought to have formed in the early universe. PBHs in the mass range between 500 trillion kg and 7 million trillion kg can constitute the entirety of dark matter. But what fraction of the observed DM density can come from PBHs of a given mass? Ranjan has made significant progress towards answering this question.
Like all black holes, PBHs produce all Standard Model particles via Hawking evaporation. The emitted positrons can annihilate with electrons in their vicinity to emit high-energy photons. Various space and balloon-based observatories have measured the electron-positron annihilation rate to great accuracy in a part of the sky – the Galactic bulge (a region around the centre of our galaxy). “If there are PBHs near the Galactic Centre, the annihilation rate of positrons from them has to be less than that recorded by the observatories,” explains Ranjan. By simply demanding that this be true, he has determined the maximum density that PBHs of a given mass can have. This then also tells us the maximum fraction of DM density that can come from them. Additionally, Ranjan has also worked with Akash Kumar Saha (an Integrated PhD student in CHEP) to study the density of PBHs from other cosmological observations.
In the future, Sudhir aims to investigate how their three-particle model of FIMPs may be tested via experiments. “Another direction is to study if our model can also explain the freeze-out mechanism for relic density,” he says. Ranjan and his group members plan to further investigate the role that PBHs can play as DM constituents.
The evidence for dark matter is both impressive and compelling. However strange it may seem, dark matter is a fundamental component of our universe, making it necessary to narrow down its composition as well as its origins. As Albert Einstein said, “The most beautiful thing we can experience is the mysterious. It is the source of all true art and all science.”