An international megaproject seeks to mimic the sun’s power to deliver clean energy
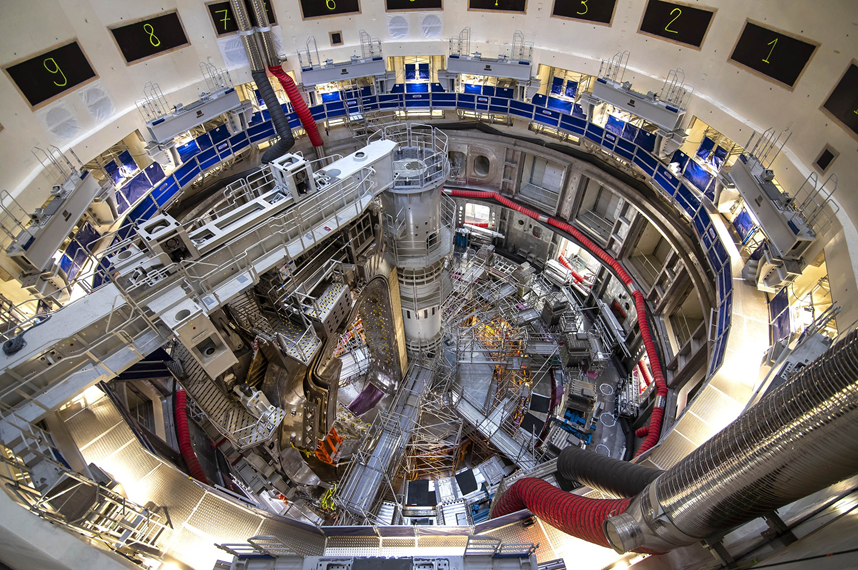
(Photo courtesy: ITER-India)
On 5 December 2022, a pellet of solid hydrogen at the National Ignition Facility (NIF) at Lawrence Livermore National Laboratory, USA, made nuclear fusion history by generating more energy than it absorbed. Using an array of high-powered lasers, researchers achieved a “burning plasma” state, generating more energy than supplied. Although meant more as a demonstration, experts consider this event a significant breakthrough in the field, and a step closer to clean and sustainable nuclear energy for all.
How is nuclear fusion different from nuclear fission, the current method used to obtain commercial-level nuclear energy? In the latter, nuclear energy is generated by breaking apart large radioactive nuclei using thermal neutrons inside a nuclear energy plant. The massive amounts of energy liberated from this nuclear splitting converts water to steam, which in turn powers turbines to generate electricity. Unlike conventional sources of energy like coal and fossil fuels, nuclear fission does not release gaseous by-products. One drawback, however, is the radioactive by-products generated as waste which must be handled and disposed of with care.
In contrast, nuclear fusion generates energy by colliding two small high-speed atoms with other atoms. Fusion occurs naturally at the heart of our sun, where hydrogen nuclei collide to produce the heat and light that makes life possible on our planet. To recreate fusion on Earth, scientists use two isotopes or forms of hydrogen – deuterium and tritium – in equal proportions and heat up this mixture to more than hundreds of millions of degrees Celsius. At these unfathomably high temperatures, the deuterium-tritium (DT) sample decomposes into a slurry of positively-charged nuclei and negatively-charged electrons – called plasma. The key to successful nuclear fusion is controlling this burning plasma.
“Presently, the entire world is focusing on two methods to confine the plasma: laser-driven fusion and magnetic confinement fusion,” explains Animesh Kuley, Assistant Professor at the Department of Physics, IISc.
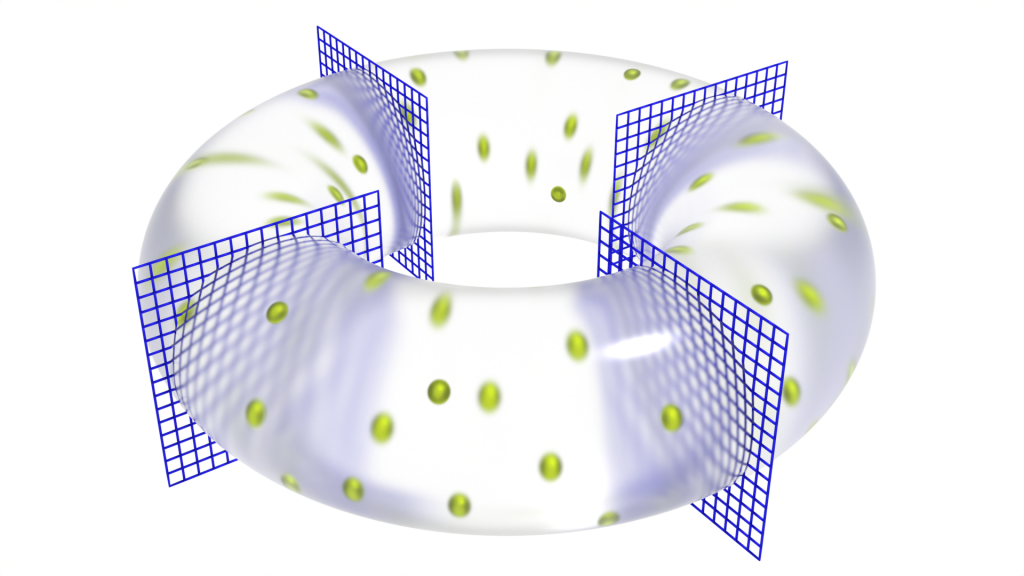
(Image: Plasma Theory Group, IISc)
In laser-driven confinement, an array of high-powered lasers is focused on a DT pellet to create a superheated plasma at the surface. This plasma ejects violently outwards, compressing the core of the pellet with its reaction force. If the ejection is strong enough, the compressed core ignites, and fusion is achieved. The NIF is the largest operational laser confinement experiment of its kind, and its recent milestone of 150% efficiency far surpasses its predecessors. But this approach is best suited for lab- or small-scale demonstrations.
On the other hand, magnetic confinement, despite working at lower efficiency, currently offers the most promise for large-scale nuclear energy production. It uses rapidly changing magnetic fields to confine and control the hot plasma, usually in a doughnut-shaped chamber called a tokamak.
The tokamak was invented in 1958 by Soviet physicists just when attempts at achieving nuclear fusion were slowing down. It now offers the most promise in achieving fusion for practical purposes. The Joint European Torus (JET), a UK-based tokamak, even made headlines in December of 2021 by creating a new fusion record, breaking their own previously set record from 24 years ago.
“The JET announced a spectacular result, I would say,” says Ujjwal Baruah, Scientist at the Institute of Plasma Research, Gandhinagar. “They generated 59 megajoules of energy for over a period of five seconds.” And although the efficiency (33%) was less compared to their 1997 record of 67%, the plasma was sustained for more than double the duration, a promising result for controlled fusion.
Ujjwal is the project director of the Indian Domestic Agency of the International Thermonuclear Energy Reactor (ITER), a massive international project aimed at building the largest experimental tokamak reactor in the world, on a 180 hectare site in France. The mission of the ITER experiment is to better understand nuclear fusion and to test technologies that would eventually be implemented in a full-scale reactor. One of the aims of this project is to achieve 10 times the energy output from the DT fusion, far surpassing the records set by both the JET and NIF, and proving the feasibility of controlled fusion.
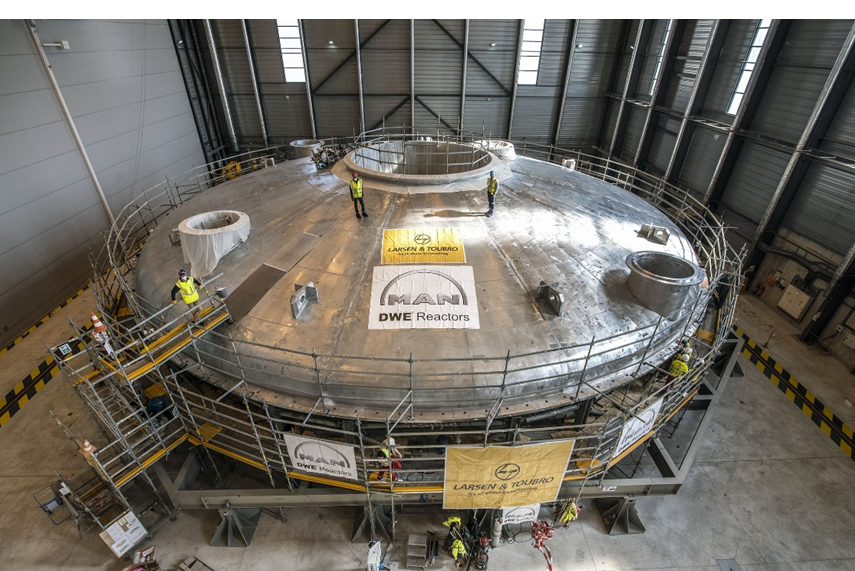
(Photo courtesy: ITER-India)
The ITER project is an aggregation of many large systems, all of which are essential to its functioning, with scientists from 38 member nations pitching in to develop them. ITER-India, led by Ujjwal, is tasked with designing the cryostat, the outer boundary of the ITER tokamak, and the water-cooling system that carries away heat from the fusion reaction. ITER-India has also designed the cryogenic distribution system that will keep the vacuum pumps and superconducting coils cool for proper functioning. These comprise the first batch of systems that ITER-India is working on – some of these systems are already being manufactured at the industrial level in India and have mostly been delivered to ITER.
The second batch of systems is less critical to the initial functioning but has specifications unique to the project. One of these is the diagnostic neutral beam system which will be used to detect the helium waste produced in the tokamak. “You cannot really purchase these systems by yourself or ask someone to engineer and deliver them,” says Ujjwal. “You need to do a lot of proof-of-principle prototyping, and a lot of fundamental R&D.” Ujjwal adds that the necessary R&D is already being carried out, and they plan to have the systems ready by the time ITER will require it, and that these “will be integrated with the other systems gradually over a period of time.”
At IISc, the Plasma Theory Group, headed by Animesh, seeks to understand plasma transport and losses in tokamaks using both theoretical tools and large-scale computer simulations. A major issue facing magnetic confinement fusion is micro-turbulence – minute instabilities in the plasma which can lead to both energy and particle loss. Using a variety of numerical modelling tools, the group can simulate this microturbulence in already existing tokamaks, such as the Large Helical Device in Japan and the ADITYA-U tokamak in India. The group has also developed their own code for modelling plasmas – called GTC-X – which focuses on plasma phenomena at various energies within the tokamak that were hitherto unexplored.
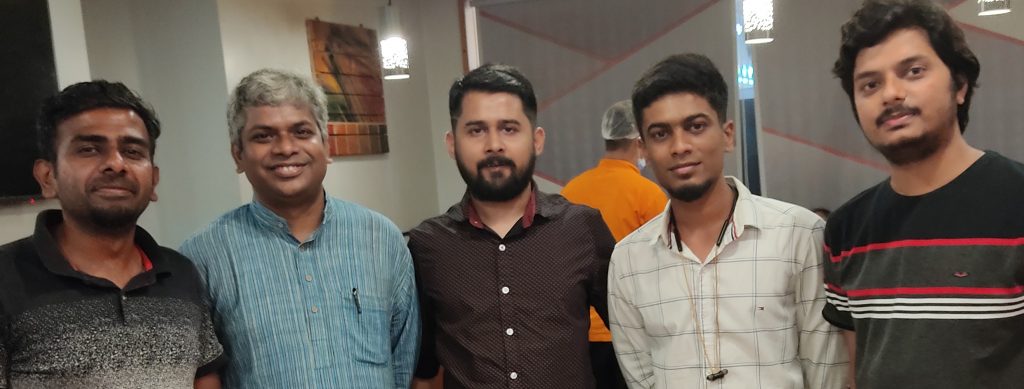
Animesh comments that many groups, such as his, are working on modelling different aspects of plasma transport as accurately as possible. He believes that when extrapolated to larger, more extensive projects such as ITER, these studies can help save “a lot of time, manpower as well as money.”
With its size and technological upgrades, ITER is guaranteed to surpass its predecessors in every aspect. However, there are still many barriers to establishing magnetic confinement fusion as a contender for conventional energy production – to power our homes and factories, for example. The primary bottleneck which ITER aims to address is control of the plasma itself. Much of the physics of how the plasma behaves inside the tokamak is not yet well understood. Plasmas are also prone to energy losses which can reduce reactor efficiency and even stop the fusion if enough heat is allowed to dissipate.
Despite these challenges, both Ujjwal and Animesh hope that the recent breakthrough at NIF spurs excitement in the nuclear fusion community, something that they believe has been lacking so far. Although plasma confinement is a primary concern, there are larger hurdles beyond: developing heat-resistant materials for the reactor, better superconductors, reliable methods for making tritium, and so on. Animesh emphasises that increased involvement of industry experts can help address these issues, in order to make fusion a reality beyond proof-of-concept.
“I see a little bit of a detachment in the Indian science community on this very important and exciting topic,” comments Ujjwal, echoing Animesh’s sentiments. “This is a very important area that has many challenges, and we need to put our brains and hands together to make it a success.”