Scientists are exploring how epigenetics drives gene expression and infection by pathogens
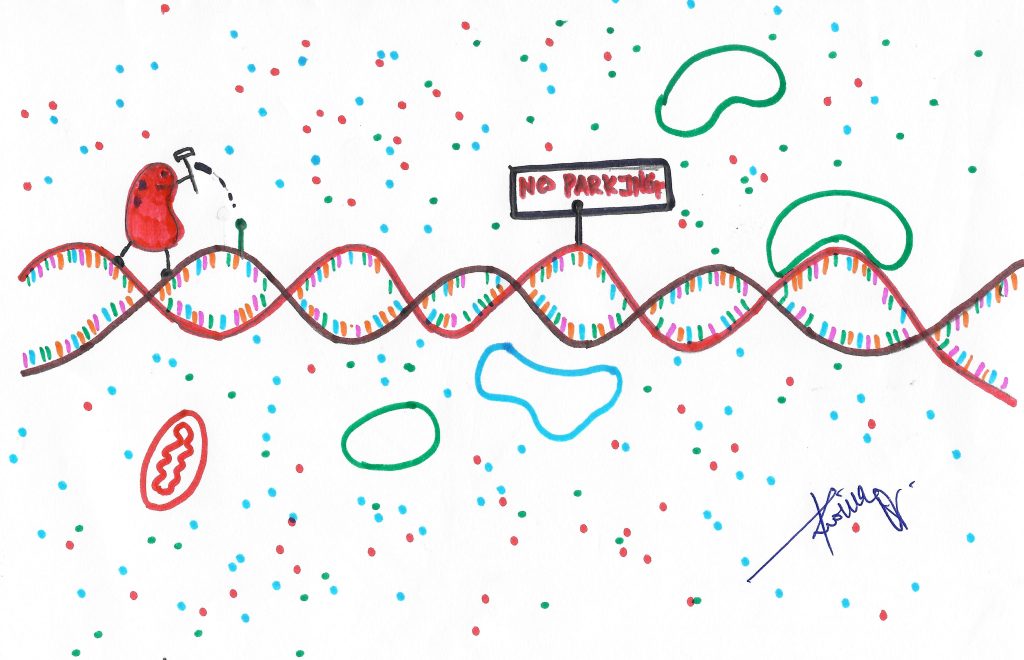
“A cell in the brain and a cell in the heart have the same DNA, but why do they have very different functions?” Meetali Singh, Assistant Professor in the Department of Developmental Biology and Genetics (DBG), posed this question to me.
This sounded similar to asking why my sambhar tastes different from my mother’s masterpiece, even though I use the same ingredients. Just like how adjusting the quantity of spices or salt can make or spoil a dish, differences in the activity of genes can change how a cell functions. And the way these changes are effected is through a phenomenon called epigenetics.
The term epigenetics literally means “above genetics,” as it pertains to processes that do not modify the DNA sequence but rather control gene expression by doing modifications on the DNA.
Each gene, when it is expressed, produces a protein. Therefore, turning off a gene means stopping the production of the protein encoded by that gene. This can be accomplished through various molecular mechanisms.
One way is to block the enzymes responsible for replicating and transcribing the gene, preventing protein expression and effectively “silencing” the gene. For example, through the process of DNA methylation, a methyl group can be attached to the promoter site of a gene, where transcription enzymes bind. This blocks the enzyme from binding and transcribing the gene. These chemical modifications on DNA are known as repressive marks.
Another approach to silence the gene is to degrade the mRNA, which serves as the template for protein synthesis following transcription. Tiny snippets called small interfering RNA (siRNA) and long noncoding RNA (lncRNA) can break down mRNA after binding to it.
Srimonta Gayen, Assistant Professor in DBG, compares this mechanism of turning a gene on and off to a switch and a bulb – we switch on the bulb only when it is required.
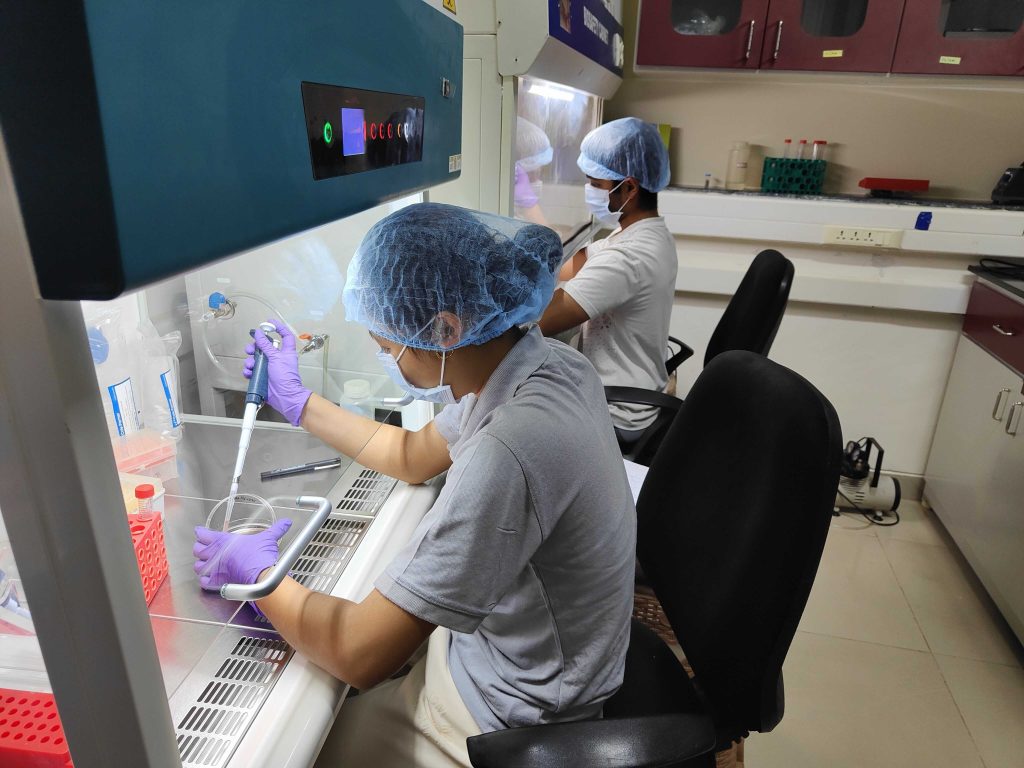
While pursuing his PhD at IIT Kharagpur, he was studying plant genetics, but a curious result from his work made him turn his attention to epigenetics. An experiment he was carrying out to breed plants involved inserting a transgene – an artificial DNA construct – into a plant. One would assume that these transgenes would also be inherited by subsequent generations. But what Srimonta noticed was that the plants did not display any physical characteristics encoded by the transgene. “So, there is another player,” he explains.
Srimonta’s lab at IISc seeks to understand how various epigenetic factors regulate cell fate specification during embryo formation and subsequent maintaining of cellular identity. By unravelling such mechanisms, his lab seeks to understand how both normal and cancer cells can be reprogrammed, which can have implications for cancer therapeutics, assisted reproduction, and improving the quality of induced pluripotent stem cells. Another major focus of his lab is how climate or environmental changes impact our epigenomes, which could lead to different diseases or health problems.
This is a hijack
Epigenetic mechanisms are key regulators of the gene expression in the cell. However, just like a plane, this gene expression can be hijacked. Some pathogens – disease-causing agents – can take control of the epigenetic mechanisms and bend the cell to their will.
Researchers in the Department of Molecular and Cell Biology (MCB), for example, study two such pathogens which can hijack the cell: Cryptococcus neoformans and Mycobacterium tuberculosis. Both pathogens can twist the epigenetic machinery of the host to establish infection using different strategies.
Cryptococcus neoformans is a fungal pathogen that causes a serious brain infection known as meningitis. It enters the body through the air, and on entering the lungs, gets ingested by immune cells called macrophages which work to eliminate foreign particles by engulfing and digesting them.
“Via epigenetics, certain pathogens including a few fungi can alter the metabolism of the macrophage by upregulating lipid metabolism genes, so that cells start synthesising more lipids. These lipids can be utilised by the pathogen for their growth and establishment of infection,” says Awantika Shah, an Integrated PhD student at MCB.
Another example involves Mycobacterium tuberculosis (Mtb), which causes tuberculosis. In a recent study, researchers identified two proteins called G9a (a methyl transferase) and Sirtuin6 (a chromatin associated protein). They found that these proteins were assisting Mtb in increasing cholesterol accumulation in the host cell, ensuring the survival of the Mtb in the hostile environment of the macrophage.
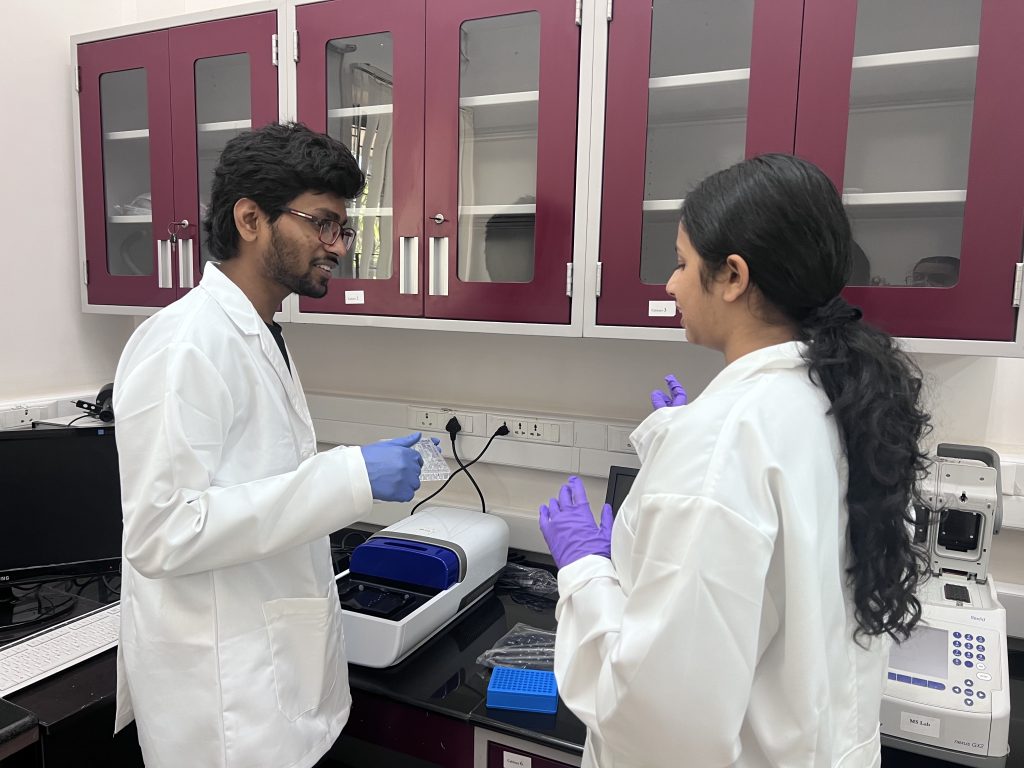
Pathogens like Mtb have been extensively studied, but there are also lesser-known protozoan parasites that are responsible for neglected tropical diseases. Meetali’s lab is focusing on such pathogens. She is particularly interested in exploring how they utilise epigenetics to facilitate their infections.
“I did my PhD work with these protozoan parasites, so they were always like the first love of my research. The second reason why I wanted to come back to this parasite is that these are evolutionarily very primitive organisms,” she explains. The evolutionary primitiveness of these pathogens will assist her in examining how modern molecular pathways like RNAi (RNA interference) evolved from their ancient ancestors in the protozoa.
One protozoan pathogen she plans to study is Toxoplasma gondii, which infects all warm-blooded animals, with the cat family members being the definitive hosts. The infection caused by Toxoplasma gondii is called toxoplasmosis.
Toxoplasmosis can be a serious condition for individuals with weakened immune systems, such as infants or pregnant women. When Toxoplasma enters the body, it is confronted by the immune system, prompting it to transform into an inactive cyst known as bradyzoite.
This transition is controlled by epigenetic mechanisms which turn on or off certain genes. One of her research goals is to understand these underlying pathways and epigenetic mechanisms, and develop strategies to control these diseases.
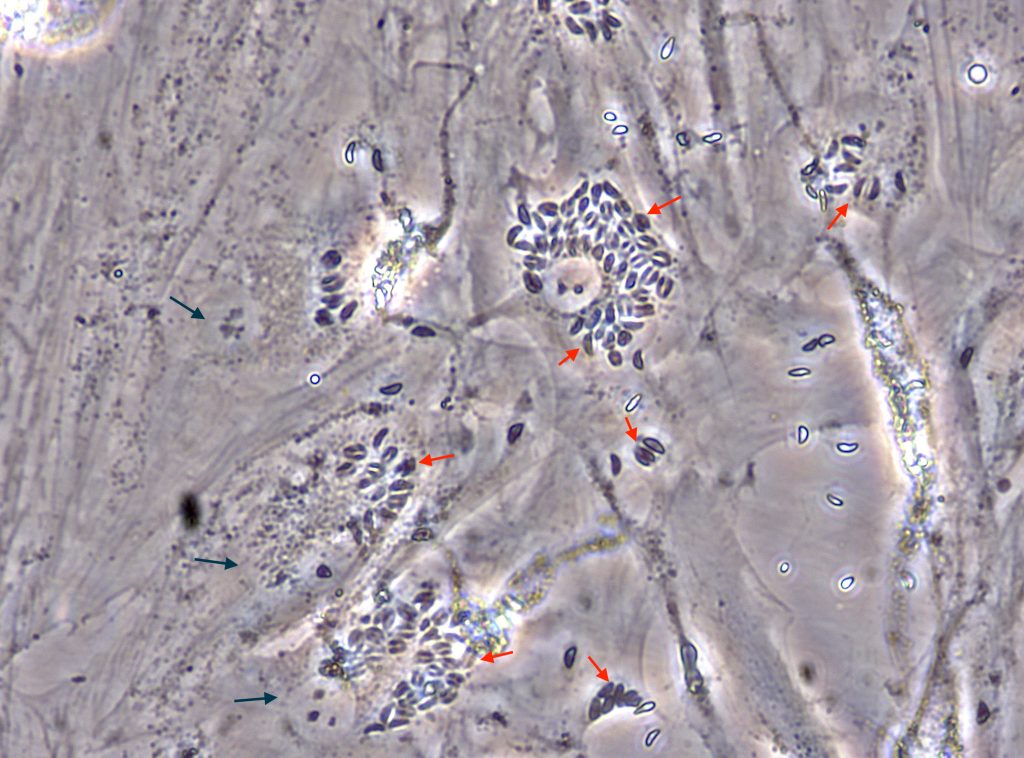
Targeting epigenetic mechanisms is increasingly becoming important for new strategies like host directed therapies (HDT), which zoom in on those cell factors that assist the pathogen in establishing the infection. For example, when G9a and Sirtuin6 were inhibited, the growth of Mtb ceased inside the macrophage cell, pointing to the potential of drugs that can act against these proteins.
“Epigenetics is also important for our normal body function,” points out Smriti Sundar, a PhD student at MCB. “So, when you are proposing a drug candidate that is going to target epigenetic factors, you need to take lots of precautions. There are lots of studies that you need to perform to ensure that it is not toxic, and it is not going to affect any normal body functions.”