Researchers at an IISc lab and start-up are working on effective vaccine strategies against two deadly viruses – SARS-CoV-2 and HIV
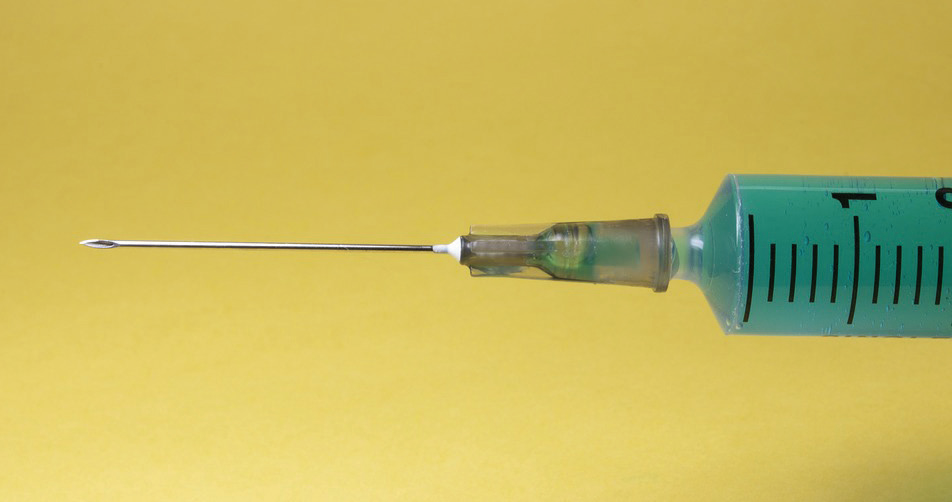
In the midst of the raging pandemic, hope bloomed this November. Two research groups announced within days of each other that their COVID-19 vaccine candidates were more than 90% effective at preventing infection. The first doses of these vaccines could be available before the end of 2020, at least in the US, if an emergency use authorisation is granted by the country’s Food and Drug Administration (FDA).
But here’s the kicker: both vaccines need to be stored and shipped at extremely cold temperatures to prevent them from losing their potency. At this point, countries like India simply do not have enough freezer boxes and supply chains to store and transport millions of doses of such vaccines, especially to far-flung corners.
A more feasible option for a country like India would be a vaccine that can be stored at room temperatures. One such heat-tolerant vaccine is currently being developed at IISc by researchers in the lab of Raghavan Varadarajan, Professor at the Molecular Biophysics Unit, and Mynvax, an IISc-incubated start-up that Varadarajan co-founded with alumnus Gautham Nadig.
In a study published recently in the Journal of Biological Chemistry, the team showed that their vaccine candidate, which triggered a strong immune response in guinea pigs, could remain stable for a month at a balmy 37°C.
Many of the 200-odd COVID-19 vaccines being developed contain the spike protein of the novel coronavirus, a molecule that sticks out of the virus’s surface. The hope is that injecting this protein, or a modified form of it, into our body will prompt our immune system to produce antibodies that may help us fight the virus if and when we get infected. But instead of the full spike protein, which needs more stringent purification protocols, Varadarajan’s team decided to use just a small portion of it – a short stretch within a region called the receptor binding domain (RBD), the part that actually binds to our cells.
First, they took the genes coding for this RBD portion and inserted it into a carrier molecule called plasmid. The plasmid was then planted into mammalian cells in the lab, which ‘read’ the genes and churned out copies of the RBD portion. These copies were then injected into guinea pigs in the lab. After a few weeks, the guinea pigs started producing antibodies against the RBD portion, enough to prevent infection. This was the expected part.
What was unexpected was that their RBD portion also turned out to be extremely sturdy – it remained active for a month at 37°C, and freeze-dried versions could handle temperatures up to 100°C. “That was a matter of luck,” says Varadarajan. The full spike protein, on the other hand, quickly lost its activity beyond 50°C.
The team will now have to carry out safety and toxicity studies in rats, along with process development and manufacture of a clinical trial batch, before they are tested in humans. “Those studies can cost about Rs 10 crore,” he says. “Unless the government funds us, we might not be able to take it forward.”
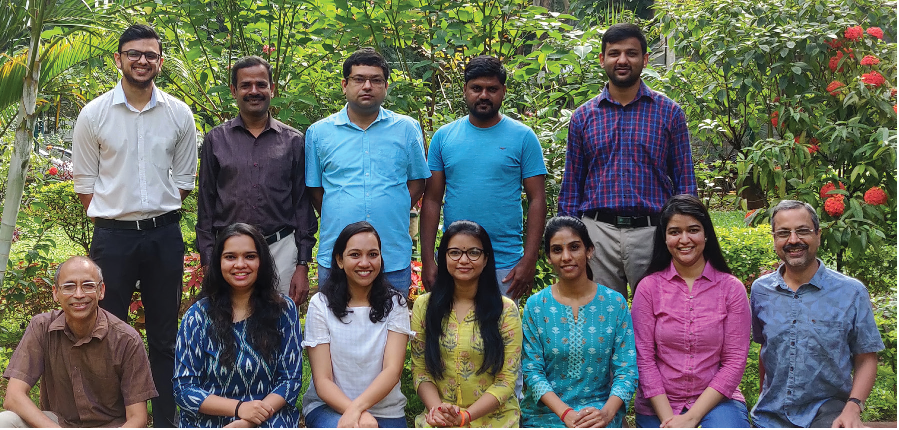
SARS-CoV-2 isn’t the only deadly virus that Varadarajan’s lab has been taking on; they have also been working on HIV. A second study published by them in the journal Proceedings of the National Academy of Sciences describes an effective method to pinpoint which parts of the HIV surface are targeted by antibodies.
Like SARS-CoV-2, HIV has an envelope protein that helps it latch on to cells in our body. Scientists have struggled to pinpoint exactly which parts of this protein – called epitopes – are targeted by neutralising antibodies, a subset of our immune system’s antibodies which can block virus entry. Vaccines based on these antibody-binding regions have a better chance of triggering a strong immune response, according to the authors.
Existing methods like X-ray crystallography and cryo-electron microscopy can help map such regions, but they are time-consuming, complicated and expensive. Cryo-electron microscopy, for example, would allow one to identify the sites where antibodies bind to, but cannot distinguish which of these are neutralising antibodies. Therefore, Varadarajan and his team decided to explore alternative approaches.
First, the team mutated the virus so that a molecule called cysteine would pop up in several places on the envelope protein. They then added a chemical label that would stick to these cysteine molecules, and finally, treated the virus with neutralising antibodies. If the antibodies could not bind to crucial sites on the virus because they were blocked by the cysteine label, the virus could survive and cause infection. These sites were then identified in one shot by sequencing the genes of the surviving mutant viruses.
“This is a rapid way of figuring out where antibodies are binding and is useful for vaccine design,” says Varadarajan. It doesn’t need expensive equipment, just gene sequencers readily accessible to most labs, he adds.
The researchers used this method to analyse the sera – the portion of blood containing mixed antibodies – from a HIV patient. “We used the sera to map unknown epitopes using the same approach,” says Rohini Datta, first author and former PhD student. “We found that the patient sera targeted two sites on the envelope protein. The fact that this sera targets these specific sites was not known before.”
Another advantage is that it can be used to identify multiple epitopes from multiple sera in parallel, she says. This could be helpful during clinical trials, for example, to see how different people’s sera react to the same vaccine.
“In principle, researchers could adapt this methodology to any virus, including SARS-CoV-2,” says Varadarajan.