Sociality in bacteria ‒ how they form aggregations, and why scientists are keen on studying them
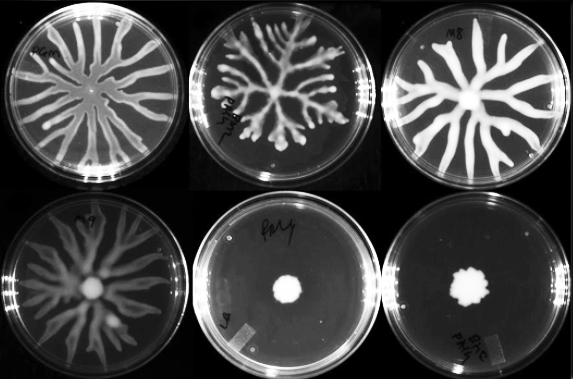
To humans, bacteria appear to lead mundane lives. These single-celled prokaryotes – so called because their cells lack a distinct nucleus and other structures – absorb nutrients from their environment, grow, and multiply on their own. But how do such relatively rudimentary organisms perform complex tasks like turn milk into yoghurt or cause diseases? The answer, at least in part, lies in the ability of several bacteria to form tightly-knit social groups in certain situations, a behaviour that has attracted the attention of many biologists, including some from IISc. Their research has led to exciting discoveries about how and why bacteria form different types of collectives.
Social bacteria
The study of social behaviour in bacteria began in 1683, when Dutch scientist Antonie van Leeuwenhoek, the world’s first microscopist, discovered a bacterial commune by studying plaque scraped off his own teeth. But it wasn’t until the 1970s that research on bacterial sociality gained traction when Danish researcher Niels Høiby showed that individuals of a bacterial species called Pseudomonas aeruginosa tend to aggregate in the lungs of patients with chronic cystic fibrosis infections. Since then, such aggregations, referred to as biofilms, have been shown to be involved in several bacterial infections in humans, and have also been documented in many other habitats.
A biofilm is formed when members of a species, or sometimes multiple species, join forces and become embedded in a slimy matrix. Bacteria produce biofilms often in response to stressful environmental conditions like a lack of moisture or nutrients, or the presence of toxins like antibiotics or disinfectants, according to Dipankar Nandi, Professor in the Department of Biochemistry at IISc. Biofilm bacteria work together to secrete the components of the slime, which protects them from unfriendly environmental conditions. They also cooperate with each other to share nutrients and remove metabolic waste.
Nandi’s lab also recently discovered a new mode of cooperation in bacteria. Alan Varghese, a former undergraduate researcher in his lab, was studying how Salmonella Typhimurium – which causes gastroenteritis in humans – responds when it is deprived of iron. During the course of his research, he stumbled upon “string-like structures” formed by these bacteria, which turned out to be a novel collective. According to Nandi, since this kind of multicellular aggregation is new to science, it was important for his team to characterise its structure in detail, and also demonstrate how they are different from classical biofilms (this bacterium also forms biofilms but under different conditions). Their discovery has just been reported in the journal Frontiers in Microbiology.
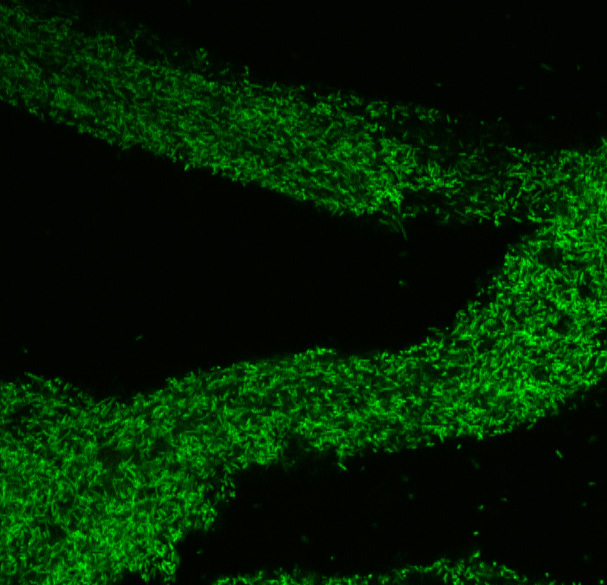
(Image courtesy: Alan Varghese, Dipankar Nandi lab)
A former Junior Research Fellow in Nandi’s lab, Samay Pande is now Assistant Professor in the Department of Microbiology and Cell Biology at IISc. For his postdoctoral research at the Institute of Integrative Biology, ETH Zurich, in Switzerland, he investigated social interactions in Myxococcus xanthus, a predatory soil bacterium. When starved, these bacteria aggregate into mounds, become spores and form a multicellular fruiting body. Upon encountering nutrients again, they germinate and start their lives afresh.
In a recent study published in Current Biology, Pande and his colleagues have demonstrated that spores of this bacterium secrete a diffusible “public good” molecule required for germination. The study also captures the flipside of sociality: conflict. In a mixed-strain fruiting body, spores belonging to a particular strain do not produce and contribute to the common pool of another important molecule needed for germination. This, according to the authors, constitutes cheating because the benefits of being in a collective accrued by these freeloaders come at a cost to other group members.
Varsha Singh, Associate Professor in the Department of Molecular Reproduction, Development and Genetics, studies another intriguing cooperative behaviour in bacteria called swarming. With the help of appendages called flagella, groups of some species move in unison in a moist medium when the situation demands. “For instance, we know that P. aeruginosa engages in swarming when a specific nutrient is limiting, such as iron or phosphate,” says Singh, who wrote a review article on the phenomenon in the Journal of the Indian Institute of Science in 2020 along with Rahul Jose, an undergraduate student in her lab. Another paper co-authored by Singh, also published last year in Physical Review E, has shown that these bacteria can also swarm away from a patch of antibiotic.
Talking bacteria
Before bacteria transform themselves from their solitary forms to their social avatars, they need to communicate with each other about whether and when to make this coordinated switch. Biologists have discovered that in order to achieve this lifestyle change, bacteria talk to each other in a chemical language. And the most popular form of chemical communication is called quorum sensing, which is seen in several bacteria, including in P. aeruginosa.
“They use a molecular signal or quorum signal to count one another. When the signal reaches a certain threshold, bacteria as a population switch their behaviour,” explains Singh. She reveals that quorum sensing in swarming P. aeruginosa is achieved with the help of a chemical called C4-homoserine lactone (C4-HSL), which can easily diffuse across the population. “When C4-HSL levels reach a threshold, the population can make a surfactant which is necessary for swarming,” she adds.
Apart from offering valuable insights into group behaviour, studying social bacteria is helping researchers explore how to make human-friendly bacteria more effective, and hostile bacteria less harmful. For instance, Samriddhi Thakur, another former undergraduate student in Nandi’s lab, has shown that biofilm formation in Salmonella could be inhibited by coumarin, a chemical that plants make to defend themselves against herbivores.
Understanding bacterial interactions in cooperatives could also address larger evolutionary questions, such as how multicellularity itself may have originated, a prospect that many biologists like Pande find exciting. “We can test social evolution hypotheses more cleanly and precisely in microbial systems,” he says.