Researchers at IISc are applying their understanding of natural processes to diverse fields
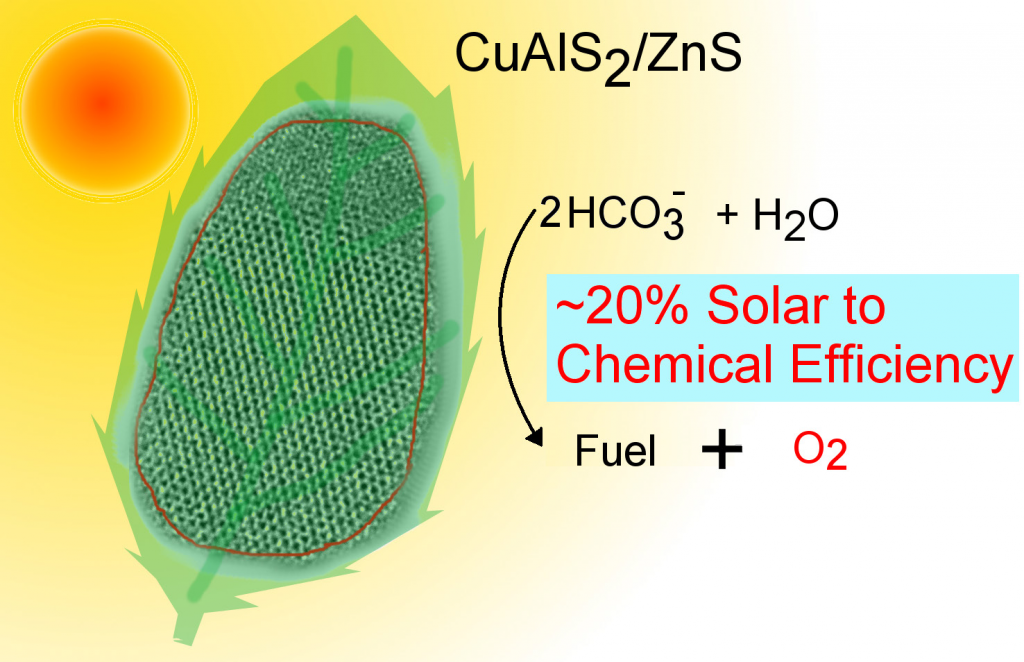
Be it the inventor of the Velcro fastener, who noticed clingy burdock seeds on his pants, or the makers of more recent ant-inspired robots that navigate better than GPS, when it comes to building things, humans have always looked to nature for inspiration. From nanomaterials to machines and architecture, scientists have long used their knowledge of biological processes to improve upon and build diverse devices and materials. Researchers at IISc too have applied their knowledge of biological processes to fields such as materials and bioinorganic chemistry, in ways that could potentially improve our understanding of biology as well.
Take photosynthesis, for example. Anshu Pandey, Associate Professor at the Solid State and Structural Chemistry Unit (SSCU), who specialises in the study of nanocrystals called quantum dots, has devised a novel way to perform artificial photosynthesis by creating a ‘quantum leaf’, along with collaborators in SSCU and the Department of Organic Chemistry. These nanocrystals can convert an inorganic carbon dioxide salt to organic fuel in the presence of visible light. Crucially, the researchers synthesised the quantum dot material (CuAlS2/ZnS) from scratch. The material has an optimal bandgap for photocatalytic light harvesting, which means that electrons can jump easily from the catalyst to nearby molecules. This property enables the material to act as a superior photocatalyst for the main reaction that produces organic carbon (in the form of formate salt) efficiently.
“The field of artificial photosynthesis has been active since the 1980s, but the real challenge has been to make a system that is efficient enough to actually be useful. Our ‘quantum leaf’ is roughly 100 times more productive than natural leaves, and the solar-to-chemical energy conversion rate is very high, at 20%,” he says. “Nature does photosynthesis in one way and does it pretty well. The goal is not simply to mimic the efficiency of living processes, but to try to exceed it through a different route. We wanted to ask if there was any other, more efficient way we can devise that serves the same utility.” The quantum leaf is currently being developed into a device for industry level use that can produce organic feedstock and fuel at a large scale.
The sun’s energy can also be harnessed through the use of solar cells. However, developing efficient solar cells is challenging, explains Praveen Ramamurthy, Professor at the Department of Materials Engineering. “There are many challenges in developing solar cells, like choosing the appropriate material and optimising device architecture. Secondly, the efficiency of such cells varies with many factors such as the angle of the incident light,” he says.
By chance, Ramamurthy and his team (comprising Jagadeesh AK, Varun A, G Hegde, and R Mahapatra) stumbled upon a discovery that biologists had made in fruit flies. These flies, which rely on sunlight for navigation like many other insects, had solved the problem quite exquisitely. Their eyes have intricate nanoscale patterns that allow them to adapt well to light-starved conditions. The grating structure of the insects’ eyes has a multiscale arrangement that absorbs light optimally by reducing the portion that is reflected in a particular wavelength.
Inspired by this design, Ramamurthy and his colleagues fabricated a silicon polystyrene architectured solar cell that performs efficiently due to the lower reflectance and the easier charge separation which is a characteristic of the material used to build the array. The team has thus built large-area solar cells with a superior and robust performance by combining biomimetics and novel material engineering. This design has made the solar cell angle of incidence agnostic, enhancing the cumulative power harvesting by about 25%.
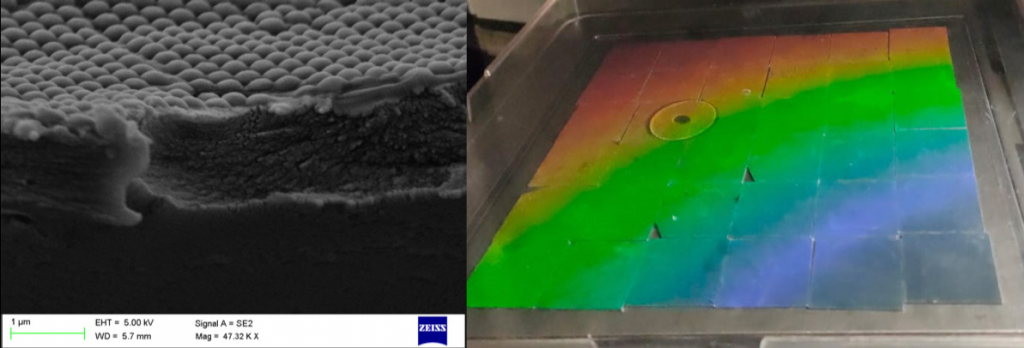
The vivid variety of living organisms around us catches our eye easily. However, there is also an invisible world at the level of cells and molecules inside our body. At this level, biological processes are built on a foundation of similar structures and biochemical reactions. All these reactions depend on catalysts called enzymes; their absence or failure could potentially lead to diseases. In recent times, researchers have been able to design enzymes in the lab tailored to perform a desired function.
But going one step further, imagine something unquestionably non living serving a living function. Enter nanozymes – bioinorganic catalysts that are small enough to pass into our cells and mimic the activity of real enzymes. G Mugesh’s group at the Department of Inorganic and Physical Chemistry works on engineering nanoscale materials that can supplement the catalytic actions of normal or dysfunctional enzymes inside our body. These actions are crucial to the smooth functioning of our tissues and organs. However, it is not an easy task to introduce any foreign substance into our body or even into single cells. It is likely that the foreign material will be rejected, either blocked by cell membranes or inactivated by chemicals secreted by our immune system.
In living cells, oxidative stress due to the presence of excess reactive oxygen species such as superoxide can damage the mitochondria and cause premature aging. Mugesh’s group has developed a cerium vanadate nanozyme which can entirely substitute the function of a metal containing enzyme called Superoxide Dismutase. It works to keep the superoxide levels in control, thereby regulating mitochondrial function in neuronal cells, and leading to an increase in the levels of a critical energy-carrying molecule called ATP. In a recent interdisciplinary study, Mugesh and collaborators also discovered that vanadium pentoxide nanosheets may successfully counter HIV-1 infection by undoing oxidative damage caused by the virus.
Some artificial enzymes even serve to fight serious infection-causing bacteria. Cerium oxide-based nanozymes synthesised via a chemical method can disrupt a process essential to cause infection: the formation of biofilms by many disease-causing bacteria like E. coli, Vibrio cholerae and Kleibsiella pneumoniae. This finding was also a collaboration between chemistry and microbiology labs. The nanozyme has been tested on urinary catheters which are susceptible to biofilm contamination in hospitals.
All these examples – the quantum leaf for photosynthesis, the fruit fly-inspired solar cell, and the artificial bioinorganic enzymes – are testimony to how knowledge can be transferred across conventional research fields and lead to unique innovations. As Ramamurthy says, “It is always a good idea to look at nature for inspiration and to not think of any problem as belonging to a specific field.”